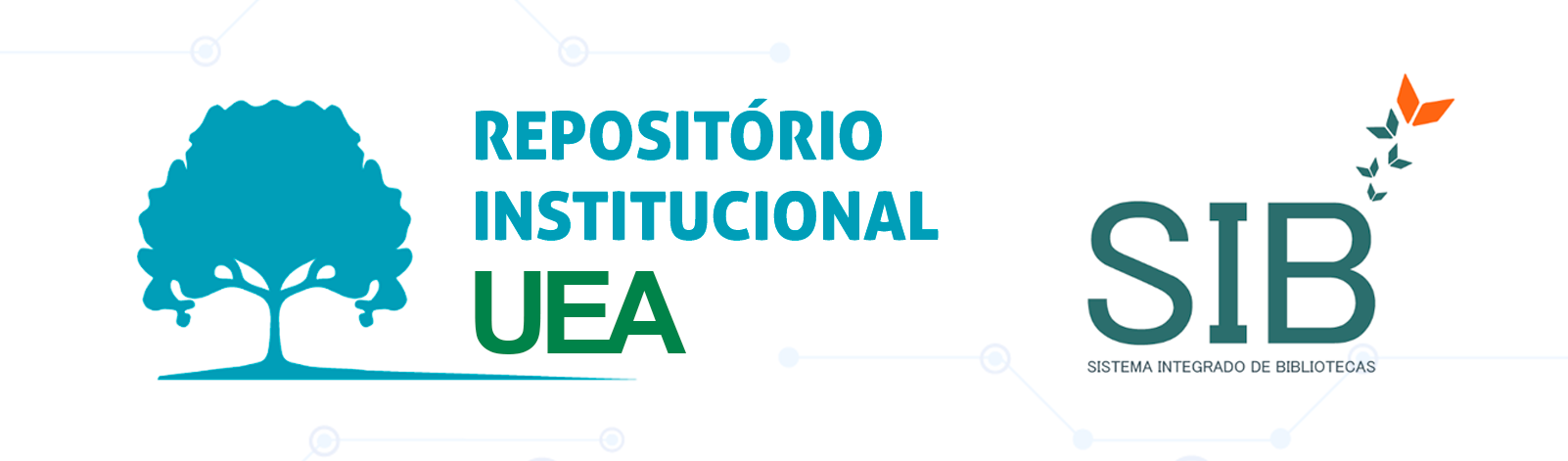
Use este identificador para citar ou linkar para este item:
http://repositorioinstitucional.uea.edu.br//handle/riuea/5345
Registro completo de metadados
Campo DC | Valor | Idioma |
---|---|---|
dc.contributor.author | Gomes, Alice de Freitas | - |
dc.date.available | 2023-11-08 | - |
dc.date.available | 2023-11-21T13:08:00Z | - |
dc.date.issued | 2023-07-16 | - |
dc.identifier.uri | http://repositorioinstitucional.uea.edu.br//handle/riuea/5345 | - |
dc.description.abstract | Leukemia represents a group of hematologic malignancies that originate from stem cells, causing the proliferation and accumulation of leukocytes in the bone marrow and blood. In 2018, leukemia was ranked the 15th most common cancer worldwide and the 11th leading cause of death. Nanoparticles as drug carriers offer advantages such as the ability to specifically target cancer cells, reduce treatment toxicity, and increase drug efficacy. The aim of this study was to evaluate the anticancer and immunomodulatory activity of bismuth nanoparticles and the nanoparticle containing crajiru (nanoparticle-CRJ) in myeloid leukemia cell lines. All tests were performed in vitro, using the HL60 (acute myeloid leukemia) and K562 (chronic myeloid leukemia) cancer cell lines, and non-cancer Vero cell lines and peripheral blood monoclonal cells (PBMC) from blood donors. The cytotoxic activity of the nanoparticles was evaluated using the MTT test, while the ability to inhibit the clonal expansion of cancer cells was investigated using the colony formation assay. To evaluate the immunomodulatory potential, pro-inflammatory and anti-inflammatory cytokines were measured by means of an immunoenzymatic assay (ELISA) in supernatant of PBMC treated with nanoparticles. The results showed that both the CRJ and Bismuth nanoparticle showed significant cytotoxic activity against the cancer cell lines HL60 (p<0.05) and K562 (p< 0.05) at all tested concentrations when compared to the control (untreated cells). No significant cytotoxic activity of the nanoparticle-CRJ was observed in non-cancer cells. The bismuth nanoparticle showed cytotoxic activity in non-cancer cells, reducing cell viability by an average of 27%, but less than that of cancer cells which reduced by an average of 43%. The bismuth nanoparticle reduced the formation of HL60 cell colonies by 79%, while the CRJ-nanoparticle reduced it by 93.95%. Regarding K562 cells, the nanoparticle-CRJ totally inhibited the formation of colonies. The nanoparticle-CRJ induced the expression of cytokines IL-6, IL-10, IL-12 and TNF-α, which demonstrates its immunomodulatory potential. The results of this study indicate that the bismuth nanoparticle has an anticancer potential, while the CRJ-nanoparticle has an anticancer and immunomodulatory potential. These data suggest that these compounds are promising candidates for prospective studies in the treatment of myeloid leukemia | pt_BR |
dc.language | por | pt_BR |
dc.publisher | Universidade do Estado do Amazonas | pt_BR |
dc.rights | Acesso Aberto | pt_BR |
dc.subject | Leucemia | pt_BR |
dc.subject | Imunomodulação | pt_BR |
dc.subject | Nanopartpiculas | pt_BR |
dc.subject | Citotoxidade | pt_BR |
dc.title | Compostos nanoparticulados : avaliação do potencial antileucêmico e imunomodulador | pt_BR |
dc.title.alternative | Nanoparticulate compounds : evaluation of antileukemic and immunomodulatory potential | pt_BR |
dc.type | Dissertação | pt_BR |
dc.date.accessioned | 2023-11-21T13:08:00Z | - |
dc.contributor.advisor-co1 | Grafov, Andriy | - |
dc.contributor.advisor-co1Lattes | http://lattes.cnpq.br/6700085891416715 | pt_BR |
dc.contributor.advisor1 | Pontes, Gemilson Soares | - |
dc.contributor.advisor1Lattes | http://lattes.cnpq.br/9081671233815990 | pt_BR |
dc.contributor.referee1 | Pontes, Gemilson Soares | - |
dc.contributor.referee1Lattes | http://lattes.cnpq.br/9081671233815990 | pt_BR |
dc.contributor.referee2 | Mariúba, Luís André Morais | - |
dc.contributor.referee2Lattes | http://lattes.cnpq.br/4784959431673419 | pt_BR |
dc.contributor.referee3 | Quintão, Jerusa Araújo | - |
dc.creator.Lattes | GOMES, A. F.;DE FREITAS GOMES, ALICE | pt_BR |
dc.description.resumo | A leucemia representa um grupo de neoplasias hematológicas que têm origem nas células-tronco, causando a proliferação e acúmulo de leucócitos na medula óssea e no sangue. Em 2018, a leucemia foi mundialmente classificada como o 15º câncer mais frequente e a 11ª principal causa de mortalidade. As nanopartículas como carreadores de medicamentos oferecem vantagens, como a capacidade de se direcionar especificamente às células cancerosas, reduzir a toxicidade do tratamento e aumentar a eficácia do medicamento. O objetivo deste estudo foi avaliar a atividade anticancerígena e imunomoduladora de nanopartículas de bismuto e a nanopartícula contendo crajiru (nanopartícula-CRJ) em linhagens celulares de leucemia mieloide. Todos os testes foram realizados in vitro, utilizando as linhagens cancerígenas HL60 (leucemia mieloide aguda) e K562 (leucemia mieloide crônica), e as células não cancerígenas da linhagem Vero e células monoclueares do sangue períferico (PBMC) de doadores de sangue. A atividade citotóxica das nanopartículas foi avaliada por meio do teste MTT, enquanto que capacidade de inibir a expansão clonal de células cancerígenas foi investigada por meio do ensaio de formação de colônias. Para avaliar o potencial imunomodulador, citocinas pro-inflamatórias e anti-inflamatórias foram dosadas por meio de um ensaio imunoenzimático (ELISA), em sobrenadante de PBMC tratadas com as nanopartículas. Os resultados demonstraram que tanto a nanopartícula-CRJ quanto a de Bismuto apresentaram atividade citotóxica significativa contra as linhagens cancerígenas HL60 (p<0.05) e K562 (p< 0.05) em todas as concentrações testadas quando comparado ao controle (células não tratadas). Não foi observada atividade citotóxica significativa da nanopartícula-CRJ em células não cancerígenas. A nanopartícula de bismuto apresentou atividade citotóxica em células não-cancerígenas, reduzindo a viabilidade das células em uma média de 27%, porém menor do que em relação às células cancerígenas que reduziram em uma média de 43%. A nanopartícula de bismuto reduziu a formação de colônias de células HL60 em 79%, enquanto que a nanopartícula-CRJ reduziu em 93,95%. Em relação às células K562, a nanopartícula-CRJ inibiu totalmente a formação de colônias. A nanopartícula-CRJ induziu a expressão das citocinas IL-6, IL-10, IL-12 e TNF-α, o que demonstra seu potencial imunomodulador. Os resultados deste estudo indicam que a nanopartícula de bismuto apresenta um potencial anticancerígeno, enquanto a nanopartícula-CRJ possui potencial antincancerígeno e imunomodulador. Estes dados sugerem que esses compostos são candidatos promissores para estudos prospectivos no tratamento de leucemia mielóide | pt_BR |
dc.publisher.country | Brasil | pt_BR |
dc.publisher.program | PPGH -PROGRAMA DE PÓS-GRADUAÇÃO EM CIÊNCIAS APLICADAS À HEMATOLOGIA | pt_BR |
dc.relation.references | 1. Sampaio MM, Santos MLC, Marques HS, Gonçalves VL de S, Araújo GRL, Lopes LW, et al. Chronic myeloid leukemia-from the Philadelphia chromosome to specific target drugs: A literature review. World J Clin Oncol. 24 de fevereiro de 2021;12(2):69–94. 2. Arber DA, Orazi A, Hasserjian R, Thiele J, Borowitz MJ, Le Beau MM, et al. The 2016 revision to the World Health Organization classification of myeloid neoplasms and acute leukemia. Blood. 19 de maio de 2016;127(20):2391–405. 3. Morales C, Cárdenas VT. Leucemia mieloide crónica: diagnóstico y tratamiento. 2010;13. 4. Hoffbrand AV, Moss PHA. Fundamentos em hematologia. 6. ed. Porto Alegre: Artmed;2018. 5. French-American-British Classification - an overview | ScienceDirect Topics [Internet]. [citado 21 de outubro de 2021]. Disponível em: https://www.sciencedirect.com/topics/medicine-and-dentistry/french-american-british-classification 6. Bray F, Ferlay J, Soerjomataram I, Siegel RL, Torre LA, Jemal A. Global cancer statistics 2018: GLOBOCAN estimates of incidence and mortality worldwide for 36 cancers in 185 countries. CA Cancer J Clin. novembro de 2018;68(6):394–424. 7. INCA. Estimativa 2020. Incidência de câncer no Brasil. Rio de Janeiro: [s.n.]. 8. de Souza Reis R, de Camargo B, de Oliveira Santos M, de Oliveira JM, Azevedo Silva F, Pombo-de-Oliveira MS. Childhood leukemia incidence in Brazil according to different geographical regions. Pediatr Blood Cancer. janeiro de 2011;56(1):58–64. 9. Reis R de S, Santos M de O, de Camargo B, Oliveira JFP, Thuler LCS, Pombo-de-Oliveira MS. Early childhood leukemia incidence trends in Brazil. Pediatr Hematol Oncol. março de 2016;33(2):83–93. 10. McNeil SE. Nanoparticle therapeutics: a personal perspective. Wiley Interdiscip Rev Nanomed Nanobiotechnol. junho de 2009;1(3):264–71. 11. Buenz EJ, Verpoorte R, Bauer BA. The Ethnopharmacologic Contribution to Bioprospecting Natural Products. Annu Rev Pharmacol Toxicol. 6 de janeiro de 2018;58:509–30. 12. Hill B. Drug resistance. Int J Oncol. 1o de agosto de 1996;9(2):197–203. 13. Mitscher LA, Pillai SP, Gentry EJ, Shankel DM. Multiple drug resistance. Med Res Rev. novembro de 1999;19(6):477–96. 14. Bharali DJ, Siddiqui IA, Adhami VM, Chamcheu JC, Aldahmash AM, Mukhtar H, et al. Nanoparticle Delivery of Natural Products in the Prevention and Treatment of Cancers: Current Status and Future Prospects. Cancers. 26 de outubro de 2011;3(4):4024–45. 52 15. Soni G, Yadav KS. Applications of nanoparticles in treatment and diagnosis of leukemia. Mater Sci Eng C Mater Biol Appl. fevereiro de 2015;47:156–64. 16. Pérez-Herrero E, Fernández-Medarde A. Advanced targeted therapies in cancer: Drug nanocarriers, the future of chemotherapy. Eur J Pharm Biopharm. 1o de junho de 2015;93:52–79. 17. Tiekink ERT. Antimony and bismuth compounds in oncology. Crit Rev Oncol Hematol. junho de 2002;42(3):217–24. 18. Tiekink ERT. Antimony and bismuth compounds in oncology. Crit Rev Oncol Hematol. junho de 2002;42(3):217–24. 19. Lima JCS, de Oliveira RG, Silva VC, de Sousa PTJr, Violante IMP, Macho A et al. Anti-inflammatory activity of 4’,6,7-trihydroxy-5-methoxyflavone from Fridericia chica (Bonpl.) LG Lohmann. Nat Prod Res 2020;34:726- 730. doi:10.1080/14786419.2018.1495636. 20. Batalha ADSJ, Souza DCM, Ubiera RD, Chaves FCM, Monteiro WM, da Silva FMA, Koolen HHF, Boechat AL, Sartim MA. Therapeutic Potential of Leaves from Fridericia chica (Bonpl.) L. G. Lohmann: Botanical Aspects, Phytochemical and Biological, Anti-Inflammatory, Antioxidant and Healing Action. Biomolecules. 2022 Aug 31;12(9):1208. doi: 10.3390/biom12091208. PMID: 36139047; PMCID: PMC9496332. 21. Moragas-Tellis CJ, Almeida-Souza F, Chagas M, Souza PVR, Silva-Silva JV, Ramos YJ et al. The Influence of anthocyanidin profile on antileishmanial activity of Arrabidaea chica morphotypes. Molecules 2020;25. doi:10.3390/molecules 25153547. 22. Velpeau A. Sur la résorption du pus et sur l’altération du sang dans les malades. Rev Med (Paris). 1827;2:216-218. 23. Piller G. Leukaemia - a brief historical review from ancient times to 1950. Br J Haematol. fevereiro de 2001;112(2):282–92. 24. Dreyfus C. Some milestones in the history of hematology. New York: Grune and Stratton 1957; 55. 25. Bennett JH. A case of hypertrophy of the spleen and liver in which death due to blood suppuration occurred. Edinb Med Surg J. 1845; 64: 413-423. 26. Virchow R . Weisses blut. Notizen de Froriep. 1845; 36 : 151-156. 27. GOODMAN LS, WINTROBE MM, DAMESHEK W, GOODMAN MJ, GILMAN A, McLENNAN MT. NITROGEN MUSTARD THERAPY: Use of Methyl-Bis(Beta-Chloroethyl)amine Hydrochloride and Tris(Beta-Chloroethyl)amine Hydrochloride for Hodgkin’s Disease, Lymphosarcoma, Leukemia and Certain Allied and Miscellaneous Disorders. J Am Med Assoc. 21 de setembro de 1946;132(3):126–32. 28. Bilieri FR, Gavinho B. A IMUNOTERAPIA PARA O TRATAMENTO DA LEUCEMIA. Rev UNIANDRADE. 30 de setembro de 2019;20(2):53–68. 53 29. Feynman R. There’s Plenty of Room at the Bottom. Em: Feynman and Computation. CRC Press; 2002. 30. Bangham AD, Standish MM, Watkins JC. Diffusion of univalent ions across the lamellae of swollen phospholipids. J Mol Biol. 1o de agosto de 1965;13(1):238-IN27. 31. Scheffel U, Rhodes BA, Natarajan TK, Wagner HN. Albumin microspheres for study of the reticuloendothelial system. J Nucl Med Off Publ Soc Nucl Med. julho de 1972;13(7):498–503. 32. Gradishar WJ, Tjulandin S, Davidson N, Shaw H, Desai N, Bhar P, et al. Phase III Trial of Nanoparticle Albumin-Bound Paclitaxel Compared With Polyethylated Castor Oil–Based Paclitaxel in Women With Breast Cancer. J Clin Oncol. 1o de novembro de 2005;23(31):7794–803. 33. Schwendener RA, Fiebig HH, Berger MR, Berger DP. Evaluation of incorporation characteristics of mitoxantrone into unilamellar liposomes and analysis of their pharmacokinetic properties, acute toxicity, and antitumor efficacy. Cancer Chemother Pharmacol. 1991;27(6):429–39. 34. Bross PF, Beitz J, Chen G, Chen XH, Duffy E, Kieffer L, et al. Approval summary: gemtuzumab ozogamicin in relapsed acute myeloid leukemia. Clin Cancer Res Off J Am Assoc Cancer Res. junho de 2001;7(6):1490–6. 35. Dinndorf PA, Gootenberg J, Cohen MH, Keegan P, Pazdur R. FDA drug approval summary: pegaspargase (oncaspar) for the first-line treatment of children with acute lymphoblastic leukemia (ALL). The Oncologist. agosto de 2007;12(8):991–8. 36. Harrison TS, Lyseng-Williamson KA. Vincristine Sulfate Liposome Injection. BioDrugs. 1o de fevereiro de 2013;27(1):69–74. 37. Krauss AC, Gao X, Li L, Manning ML, Patel P, Fu W, et al. FDA Approval Summary: (Daunorubicin and Cytarabine) Liposome for Injection for the Treatment of Adults with High-Risk Acute Myeloid Leukemia. Clin Cancer Res Off J Am Assoc Cancer Res. 1o de maio de 2019;25(9):2685–90. 38. Tebbi CK. Etiology of Acute Leukemia: A Review. Cancers. janeiro de 2021;13(9):2256. 39. Stieglitz E, Loh ML. Genetic predispositions to childhood leukemia. Ther Adv Hematol. agosto de 2013;4(4):270–90. 40. De Kouchkovsky I, Abdul-Hay M. “Acute myeloid leukemia: a comprehensive review and 2016 update”. Blood Cancer J. 1o de julho de 2016;6(7):e441. 41. Abreu GM, Sousa SC de, Gomes EV. Leucemia Linfoide e Mieloide: Uma breve revisão narrativa / Lymphoid and Myeloid Leukemia: A brief narrative review. Braz J Dev. 13 de agosto de 2021;7(8):80666–81. 42. Meenaghan T, Dowling M, Kelly M. Acute leukaemia: making sense of a complex blood cancer. Br J Nurs Mark Allen Publ. 26 de fevereiro de 2012;21(2):76, 78–83. 54 43. Gralnick HR, Galton D a. G, Catovsky D, Sultan C, Bennett JM. Classification of Acute Leukemia. Ann Intern Med. 1o de dezembro de 1977;87(6):740–53. 44. SEER [Internet]. [citado 24 de setembro de 2021]. Leukemia - Cancer Stat Facts. Disponível em: https://seer.cancer.gov/statfacts/html/leuks.html 45. Noone A , Howlader N , Krapcho M , Miller D , Brest A , Yu M , Ruhl J , Tatalovich Z , Mariotto A , Lewis D , et al. SEER Cancer Statistics Review, 1975 - 2015 . Instituto Nacional do Câncer , Bethesda , Maryland. 2017. 46. Bispo JAB, Pinheiro PS, Kobetz EK. Epidemiology and Etiology of Leukemia and Lymphoma. Cold Spring Harb Perspect Med. 1o de junho de 2020;10(6):a034819. 47. Siegel DA, Henley SJ, Li J, Pollack LA, Dyne EAV, White A. Rates and Trends of Pediatric Acute Lymphoblastic Leukemia — United States, 2001–2014. MMWR Morb Mortal Wkly Rep. 15 de setembro de 2017;66(36):950. 48. Gouveia M de S, Batista JKM, Passos TS, Prado BS, Siqueira CE, Almeida-Santos MA. Comparison of factors associated with leukemia and lymphoma mortality in Brazil. Cad Saúde Pública [Internet]. 3 de agosto de 2020 [citado 24 de setembro de 2021];36. Disponível em: http://www.scielo.br/j/csp/a/xLqHBXqZPR4WGkRSxWcctyb/?lang=en 49. Silva-Junior AL, Alves FS, Kerr MWA, Xabregas LA, Gama FM, Rodrigues MGA, et al. Acute lymphoid and myeloid leukemia in a Brazilian Amazon population: Epidemiology and predictors of comorbidity and deaths. PloS One. 2019;14(8):e0221518. 50. Ho MSH, Medcalf RL, Livesey SA, Traianedes K. The dynamics of adult haematopoiesis in the bone and bone marrow environment. Br J Haematol. agosto de 2015;170(4):472–86. 51. Rose-Inman H, Kuehl D. Acute Leukemia. Emerg Med Clin North Am. 1o de agosto de 2014;32(3):579–96. 52. Lyengar, V., & Shimanovsky, A. (2021). Leukemia. In StatPearls. StatPearls Publishing. 53. Ma Y, Dobbins SE, Sherborne AL, Chubb D, Galbiati M, Cazzaniga G, et al. Developmental timing of mutations revealed by whole-genome sequencing of twins with acute lymphoblastic leukemia. Proc Natl Acad Sci U S A. 30 de abril de 2013;110(18):7429–33. 54. Moorman AV, Ensor HM, Richards SM, Chilton L, Schwab C, Kinsey SE, et al. Prognostic effect of chromosomal abnormalities in childhood B-cell precursor acute lymphoblastic leukaemia: results from the UK Medical Research Council ALL97/99 randomised trial. Lancet Oncol. maio de 2010;11(5):429–38. 55. Kang ZJ, Liu YF, Xu LZ, Long ZJ, Huang D, Yang Y, et al. The Philadelphia chromosome in leukemogenesis. Chin J Cancer. 27 de maio de 2016;35:48. 56. INCA - Instituto Nacional de Câncer [Internet]. 2018 [citado 24 de setembro de 2021]. Leucemia. Disponível em: https://www.inca.gov.br/tipos-de-cancer/leucemia 57. Severson C. Hematologic Malignancies in Adults. Can Oncol Nurs J. 1o de maio de 2016;26(2):177. 55 58. Jabbour E, Cortes JE, Kantarjian HM. Molecular monitoring in chronic myeloid leukemia: response to tyrosine kinase inhibitors and prognostic implications. Cancer. 15 de maio de 2008;112(10):2112–8. 59. Hills RK, Castaigne S, Appelbaum FR, Delaunay J, Petersdorf S, Othus M, et al. Addition of gemtuzumab ozogamicin to induction chemotherapy in adult patients with acute myeloid leukaemia: a meta-analysis of individual patient data from randomised controlled trials. Lancet Oncol. 1o de agosto de 2014;15(9):986–96. 60. Bolin RW, Robinson WA, Sutherland J, Hamman RF. Busulfan versus hydroxyurea in long-term therapy of chronic myelogenous leukemia. Cancer. 1982;50(9):1683–6. 61. Blackburn LM, Bender S, Brown S. Acute Leukemia: Diagnosis and Treatment. Semin Oncol Nurs. 1o de dezembro de 2019;35(6):150950. 62. Chronic lymphocytic leukemia: 2017 update on diagnosis, risk stratification, and treatment - Hallek - 2017 - American Journal of Hematology - Wiley Online Library [Internet]. [citado 25 de setembro de 2021]. Disponível em: https://onlinelibrary.wiley.com/doi/10.1002/ajh.24826 63. Rogers PC, Melnick SJ, Ladas EJ, Halton J, Baillargeon J, Sacks N. Children’s Oncology Group (COG) Nutrition Committee. Pediatr Blood Cancer. 2008;50(S2):447–50. 64. Adebayo IA, Usman AI, Shittu FB, Ismail NZ, Arsad H, Muftaudeen TK, Samian MR. Boswellia dalzielii-Mediated Silver Nanoparticles Inhibited Acute Myeloid Leukemia (AML) Kasumi-1 Cells by Inducing Cell Cycle Arrest. Bioinorg Chem Appl. 2020 Sep 22;2020:8898360. doi: 10.1155/2020/8898360. PMID: 33029114; PMCID: PMC7528135. 65. Bodet-Milin C, Kraeber-Bodéré F, Eugène T, Guérard F, Gaschet J, Bailly C, et al. Radioimmunotherapy for Treatment of Acute Leukemia. Semin Nucl Med. 1o de março de 2016;46(2):135–46. 66. Safety of Yttrium-90 Ibritumomab Tiuxetan Radioimmunotherapy for Relapsed Low-Grade, Follicular, or Transformed Non-Hodgkin’s Lymphoma | Journal of Clinical Oncology [Internet]. [citado 29 de setembro de 2021]. Disponível em: https://ascopubs.org/doi/10.1200/JCO.2003.08.043 67. Tan S, Li D, Zhu X. Cancer immunotherapy: Pros, cons and beyond. Biomed Pharmacother. 1o de abril de 2020;124:109821. 68. Kroschinsky F, Stölzel F, von Bonin S, Beutel G, Kochanek M, Kiehl M, et al. New drugs, new toxicities: severe side effects of modern targeted and immunotherapy of cancer and their management. Crit Care. 14 de abril de 2017;21(1):89. 69. Svenberg P, Remberger M, Svennilson J, Mattsson J, Leblanc K, Gustafsson B, et al. Allogenic stem cell transplantation for nonmalignant disorders using matched unrelated donors. Biol Blood Marrow Transplant. 1o de dezembro de 2004;10(12):877–82. 70. Longley D, Johnston P. Molecular mechanisms of drug resistance. J Pathol. 2005;205(2):275–92. 56 71. Kartal-Yandim M, Adan-Gokbulut A, Baran Y. Molecular mechanisms of drug resistance and its reversal in cancer. Crit Rev Biotechnol. 3 de julho de 2016;36(4):716–26. 72. Huh HJ, Park CJ, Jang S, Seo EJ, Chi HS, Lee JH, et al. Prognostic Significance of Multidrug Resistance Gene 1 (MDR1), Multidrug Resistance-related Protein (MRP) and Lung Resistance Protein (LRP) mRNA Expression in Acute Leukemia. J Korean Med Sci. abril de 2006;21(2):253–8. 73. Follini E, Marchesini M, Roti G. Strategies to Overcome Resistance Mechanisms in T-Cell Acute Lymphoblastic Leukemia. Int J Mol Sci. 20 de junho de 2019;20(12):3021. 74. Auberger P, Tamburini-Bonnefoy J, Puissant A. Drug Resistance in Hematological Malignancies. Int J Mol Sci. janeiro de 2020;21(17):6091. 75. Alfarouk KO, Stock CM, Taylor S, Walsh M, Muddathir AK, Verduzco D, et al. Resistance to cancer chemotherapy: failure in drug response from ADME to P-gp. Cancer Cell Int. 2015;15:71. 76. Krishna R, Mayer LD. Multidrug resistance (MDR) in cancer. Mechanisms, reversal using modulators of MDR and the role of MDR modulators in influencing the pharmacokinetics of anticancer drugs. Eur J Pharm Sci Off J Eur Fed Pharm Sci. outubro de 2000;11(4):265–83. 77. Vauthier C, Dubernet C, Chauvierre C, Brigger I, Couvreur P. Drug delivery to resistant tumors: the potential of poly(alkyl cyanoacrylate) nanoparticles. J Control Release Off J Control Release Soc. 5 de dezembro de 2003;93(2):151–60. 78. Kirtane AR, Kalscheuer SM, Panyam J. Exploiting nanotechnology to overcome tumor drug resistance: Challenges and opportunities. Adv Drug Deliv Rev. 30 de novembro de 2013;65(13):1731–47. 79. Bea DB, Tejeda AP, Pardo AA, et al. Nanomedicine: general aspects of a promissory future. R e v Habanera Cienc Méd. 2011;10(3):410-21. 80. Farokhzad OC, Langer R. Impact of Nanotechnology on Drug Delivery. ACS Nano. 27 de janeiro de 2009;3(1):16–20. 81. Abriata JP, Eloy JO, Riul TB, Campos PM, Baruffi MD, Marchetti JM. Poly-epsilon-caprolactone nanoparticles enhance ursolic acid in vivo efficacy against Trypanosoma cruzi infection. Mater Sci Eng C Mater Biol Appl. 1o de agosto de 2017;77:1196–203. 82. Hare JI, Lammers T, Ashford MB, Puri S, Storm G, Barry ST. Challenges and strategies in anti-cancer nanomedicine development: An industry perspective. Adv Drug Deliv Rev. 1o de janeiro de 2017;108:25–38. 83. Asai T. Nanoparticle-mediated delivery of anticancer agents to tumor angiogenic vessels. Biol Pharm Bull. 2012;35(11):1855–61. 84. Hasan S. (2015). A review on nanoparticles: their synthesis and types. Res. J. Recent Sci. 4, 1–3. [Google Scholar]. 57 85. Mendes R., Carreira B., Baptista P. V., Fernandes A. R. (2016). Non-small cell lung cancer biomarkers and targeted therapy – two faces of the same coin fostered by nanotechnology. Expert Rev. Precis. Med. Drug Dev. 1, 155–168. 10.1080/23808993.2016.1159914 [CrossRef] [Google Scholar] [Ref list]. 86. Wang L, Wang Z, Cao L, Ge K. Constructive strategies for drug delivery systems in antivirus disease therapy by biosafety materials. Biosaf Health. 2022 Jun;4(3):161-170. doi: 10.1016/j.bsheal.2022.03.008. Epub 2022 Mar 11. PMID: 35291339; PMCID: PMC8912974. 87. G. Barratt Colloidal drug carriers: achievements and perspectives Cell. Mol. Life Sci., 60 (2003), pp. 21-37. 88. Mundekkad D, Cho WC. Nanoparticles in Clinical Translation for Cancer Therapy. Int J Mol Sci. 2022 Feb 1;23(3):1685. doi: 10.3390/ijms23031685. PMID: 35163607; PMCID: PMC8835852. 89. ZnO Nanoparticles: A Promising Anticancer Agent - Gunjan Bisht, Sagar Rayamajhi, 2016 [Internet]. [citado 29 de setembro de 2021]. Disponível em: https://journals.sagepub.com/doi/10.5772/63437 90. Dual functions of silver nanoparticles in F9 teratocarcinoma stem cell | IJN [Internet]. [citado 29 de setembro de 2021]. Disponível em: https://www.dovepress.com/dual-functions-of-silver-nanoparticles-in-f9-teratocarcinoma-stem-cell-peer-reviewed-fulltext-article-IJN 91. Protein Nanoparticles as Drug Delivery Carriers for Cancer Therapy [Internet]. [citado 29 de setembro de 2021]. Disponível em: https://www.hindawi.com/journals/bmri/2014/180549/ 92. Chung IM, Abdul Rahuman A, Marimuthu S, Vishnu Kirthi A, Anbarasan K, Rajakumar G. An Investigation of the Cytotoxicity and Caspase-Mediated Apoptotic Effect of Green Synthesized Zinc Oxide Nanoparticles Using Eclipta prostrata on Human Liver Carcinoma Cells. Nanomaterials. 12 de agosto de 2015;5(3):1317–30. 93. Lee MJ, Lee SJ, Yun SJ, Jang JY, Kang H, Kim K, et al. Silver nanoparticles affect glucose metabolism in hepatoma cells through production of reactive oxygen species. Int J Nanomedicine. 22 de dezembro de 2015;11:55–68. 94. Wong HL, Bendayan R, Rauth AM, Xue HY, Babakhanian K, Wu XY. A mechanistic study of enhanced doxorubicin uptake and retention in multidrug resistant breast cancer cells using a polymer-lipid hybrid nanoparticle system. J Pharmacol Exp Ther. junho de 2006;317(3):1372–81. 95. Khawar IA, Kim JH, Kuh HJ. Improving drug delivery to solid tumors: priming the tumor microenvironment. J Control Release Off J Control Release Soc. 10 de março de 2015;201:78–89. 96. Tomasina J, Lheureux S, Gauduchon P, Rault S, Malzert-Fréon A. Nanocarriers for the targeted treatment of ovarian cancers. Biomaterials. janeiro de 2013;34(4):1073–101. 58 97. Sriraman S. K., Aryasomayajula B., Torchilin V. P. (2014). Barriers to drug delivery in solid tumors. Tissue Barriers 2, e29528. 10.4161/tisb.29528 [PMC free article] [PubMed] [CrossRef] [Google Scholar] [Ref list]. 98. Panyam J, Labhasetwar V. Sustained cytoplasmic delivery of drugs with intracellular receptors using biodegradable nanoparticles. Mol Pharm. 12 de janeiro de 2004;1(1):77–84. 99. Yadav KS, Sawant KK. Formulation optimization of etoposide loaded PLGA nanoparticles by double factorial design and their evaluation. Curr Drug Deliv. janeiro de 2010;7(1):51–64. 100. Yadav KS, Sawant KK. Modified Nanoprecipitation Method for Preparation of Cytarabine-Loaded PLGA Nanoparticles. AAPS PharmSciTech. 1o de setembro de 2010;11(3):1456–65. 101. Ahmad S, Isab AA, Ali S, Al-Arfaj AR. Perspectives in bioinorganic chemistry of some metal based therapeutic agents. Polyhedron. 8 de maio de 2006;25(7):1633–45. 102. Huang R, Wallqvist A, Covell DG. Anticancer metal compounds in NCI’s tumor-screening database: putative mode of action. Biochem Pharmacol. 1o de abril de 2005;69(7):1009–39. 103. Marzano IM, Tomco D, Staples RJ, Lizarazo-Jaimes EH, Gomes DA, Bucciarelli-Rodriguez M, et al. Dual anticancer and antibacterial activities of bismuth compounds based on asymmetric [NN’O] ligands. J Inorg Biochem. setembro de 2021;222:111522. 104. Cabral-Romero C, Solís-Soto JM, Sánchez-Pérez Y, et al. Antitumor activity of a hydrogel loaded with lipophilic bismuth nanoparticles on cervical, prostate, and colon human cancer cells. Anticancer Drugs. 2020;31(3):251-259. doi:10.1097/CAD.0000000000000863. 105. Ribeiro AFC, Telles TC, Ferraz VP, Souza-Fagundes EM, Cassali GD, Carvalho AT et al. Effect of Arrabidaea chica extracts on the ehrlich solid tumor development. Revista Brasileira de Farmacognosia 2012;22:364-373. 106. Rodrigues M, Muehlmann L, Longo J, Silva R, Graebner I, Degterev I, et al. Photodynamic Therapy Based on Arrabidaea chica (Crajiru) Extract Nanoemulsion: In vitro Activity against Monolayers and Spheroids of Human Mammary Adenocarcinoma MCF-7 Cells. J Nanomedicine Nanotechnol. 1o de janeiro de 2015;06. 107. Chen C, Lv G, Pan C, Song M, Wu C, Guo D, et al. Poly(lactic acid) (PLA) based nanocomposites—a novel way of drug-releasing. Biomed Mater. setembro de 2007;2(4):L1–4. 108. Fan L, Liu C, Hu A, Liang J, Li F, Xiong Y, et al. Dual oligopeptides modification mediates arsenic trioxide containing nanoparticles to eliminate primitive chronic myeloid leukemia cells inside bone marrow niches. Int J Pharm. 15 de abril de 2020;579:119179. 109. Yadav KS, Jacob S, Sachdeva G, Sawant KK. Intracellular Delivery of Etoposide Loaded Biodegradable Nanoparticles: Cytotoxicity and Cellular Uptake Studies. J Nanosci Nanotechnol. 1o de agosto de 2011;11(8):6657–67. 59 110. Longley D, Johnston P. Molecular mechanisms of drug resistance. J Pathol. 2005;205(2):275–92. 111. Kartal-Yandim M, Adan-Gokbulut A, Baran Y. Molecular mechanisms of drug resistance and its reversal in cancer. Crit Rev Biotechnol. 3 de julho de 2016;36(4):716–26. 112. Huh HJ, Park C-J, Jang S, Seo E-J, Chi H-S, Lee J-H, et al. Prognostic Significance of Multidrug Resistance Gene 1 (MDR1), Multidrug Resistance-related Protein (MRP) and Lung Resistance Protein (LRP) mRNA Expression in Acute Leukemia. J Korean Med Sci. abril de 2006;21(2):253–8. 113. FESSI, H.; PUISIEUX, F.; DEVISSAGUET, J.P.; AMMOURY, N.; BENITA, S. Nanocapsule formation by interfacial polymer deposition following solvent displacement. Int. J. Pharm., v. 55, p. R1–R4, 1989. 114. FLORENTINO NETO, S., PRADA, A. L., ACHOD, L. D. R., TORQUATO, H. F. V., LIMA, C. S., PAREDES-GAMERO, E. J., T.P. SOUZA. AMADO, J. R. R. (2021). α-amyrin-loaded nanocapsules produce selective cytotoxic activity in leukemic cells. Biomedicine & Pharmacotherapy, 139, 111656. 115. Oliveira R, Pontes G, Kostyuk A, Camargo G, Dhyani A, Shvydenko T, et al. Anticancer and Immunomodulatory Activities of a Novel Water-Soluble Derivative of Ellipticine. Molecules. 1o de maio de 2020;25:2130. 116. Cuimei Liu, Lingyu Zhang, Xiangjun Chen, Shengnan Li, Qinghe Han, Lu Li, Chungang Wang, Biomolecules-assisted synthesis of degradable bismuth nanoparticles for dual-modal imaging-guided chemo-photothermal therapy, Chemical Engineering Journal, Volume 382, 2020, 122720, ISSN 1385-8947, https://doi.org/10.1016/j.cej.2019.122720. (https://www.sciencedirect.com/science/article/pii/S1385894719321230). 117. Ahamed M, Akhtar MJ, Khan MAM, Alaizeri ZM, Alhadlaq H. Facile Synthesis of Zn-Doped Bi2O3 Nanoparticles and Their Selective Cytotoxicity toward Cancer Cells. ACS Omega. 2021 Jun 29;6(27):17353-17361. doi: 10.1021/acsomega.1c01467. PMID: 34278121; PMCID: PMC8280700. 118. Huang R, Wallqvist A, Covell DG. Anticancer metal compounds in NCI’s tumorscreening database: putative mode of action. Biochem Pharmacol. 1o de abril de 2005;69(7):1009–39. 119. Marzano IM, Tomco D, Staples RJ, Lizarazo-Jaimes EH, Gomes DA, BucciarelliRodriguez M, et al. Dual anticancer and antibacterial activities of bismuth compounds based on asymmetric [NN’O] ligands. J Inorg Biochem. setembro de 2021;222:111522. 120. Hernandez-Delgadillo R, García-Cuéllar CM, Sánchez-Pérez Y, Pineda-Aguilar N, Martínez-Martínez MA, Rangel-Padilla EE, Nakagoshi-Cepeda SE, Solís-Soto JM, Sánchez-Nájera RI, Nakagoshi-Cepeda MAA, Chellam S, Cabral-Romero C. In vitro evaluation of the antitumor effect of bismuth lipophilic nanoparticles (BisBAL NPs) on breast cancer cells. Int J Nanomedicine. 2018 Oct 5;13:6089-6097. doi: 10.2147/IJN.S179095. PMID: 30323596; PMCID: PMC6179729. 60 121. Denise Taffarello, Michelle P. Jorge, Ilza Ma de O. Sousa, Marta C. T. Duarte, Glyn M. Figueira, Nubia de C. A. Queiroz, Rodney A. F. Rodrigues, Joao E. de Carvalho, Ana L. T. R. Goes, Mary A. Foglio, José M. Riveros, Marcos N. Eberlin e Elaine C. CabralATIVIDADE DE EXTRATOS DE Arrabidaea chica (HUMB. & BONPL.) VERLOT OBTIDOS POR PROCESSOS BIOTECNOLÓGICOS SOBRE A PROLIFERAÇÃO DE FIBROBLASTOS E CÉLULAS TUMORAIS HUMANASQuim. Nova, Vol. 36, No. 3, 431-436, 2013. 122. Servat-Medina L, González-Gómez A, Reyes-Ortega F, Sousa IM, Queiroz Nde C, Zago PM, Jorge MP, Monteiro KM, de Carvalho JE, San Román J, Foglio MA. Chitosan-tripolyphosphate nanoparticles as Arrabidaea chica standardized extract carrier: synthesis, characterization, biocompatibility, and antiulcerogenic activity. Int J Nanomedicine. 2015 Jun 9;10:3897-909. doi: 10.2147/IJN.S83705. PMID: 26089666; PMCID: PMC4467739. 123. Wahid B, Ali A, Rafique S, Waqar M, Wasim M, Wahid K, Idrees M. An overview of cancer immunotherapeutic strategies. Immunotherapy 2018, 10, 999–1010. [CrossRef] [PubMed]. 124. Weiden J, Tel J, Figdor C G. Synthetic immune niches for cancer immunotherapy. Nat. Rev. Immunol. 2018, 18, 2012–2019. [CrossRef] [PubMed]. 125. Shurin, M. Cancer as an immune-mediated disease. ImmunoTargetsTher. 2012, 1, 1–6. [CrossRef] [PubMed]. 126. Mikucki, M.E.; Fisher, D.T.; Ku, A.W.; Appenheimer, M.M.; Muhitch, J.B.; Evans, S.S. Preconditioning thermal therapy: flipping the switch on IL-6 for anti-tumour immunity. Int. J. Hyperth. Off. J. Eur. Soc. Hyperthermic Oncol. North Am. Hyperth. Gr. 2013, 29, 464–473, doi:10.3109/02656736.2013.807440. 127. Fisher, D.T.; Chen, Q.; Skitzki, J.J.; Muhitch, J.B.; Zhou, L.; Appenheimer, M.M.; Vardam, T.D.; Weis, E.L.; Passanese, J.; Wang, W.-C.; et al. IL-6 trans-signaling licenses mouse and human tumor microvascular gateways for trafficking of cytotoxic T cells. J. Clin. Invest. 2011, 121, 3846–3859, doi:10.1172/JCI44952. 128. Grivennikov SI, Greten F R, Karin M. Immunity, Inflammation, and Cancer. Cell 2010, 140, 883–899. [CrossRef]. 129. Schreiber RD, Old LJ, Smyth MJ. Cancer immunoediting: Integrating immunity’s roles in cancer suppression and promotion. Science 2011, 331, 1565–1570. [CrossRef]. 130. Fisher DT, Appenheimer MM, Evans SS. The two faces of IL-6 in the tumor microenvironment. Semin. Immunol. 2014, 26, 38–47. [CrossRef]. 131. Oft, M. IL-10: master switch from tumor-promoting inflammation to antitumor immunity. Cancer Immunol. Res. 2014, 2, 194–199, doi:10.1158/2326-6066.CIR-13-0214. 132. Lu, X. Impact of IL-12 in Cancer. Curr. Cancer Drug Targets 2017, 17, 682–697, doi:10.2174/1568009617666170427102729. 133. Nguyen, K.G.; Vrabel, M.R.; Mantooth, S.M.; Hopkins, J.J.; Wagner, E.S.; Gabaldon, T.A.; Zaharoff, D.A. Localized Interleukin-12 for Cancer Immunotherapy. Front. Immunol. 2020, 11, 575597, doi:10.3389/fimmu.2020.575597. 61 134. Josephs, S.F.; Ichim, T.E.; Prince, S.M.; Kesari, S.; Marincola, F.M.; Escobedo, A.R.; Jafri, A. Unleashing endogenous TNF-alpha as a cancer immunotherapeutic. J. Transl. Med. 2018, 16, 242, doi:10.1186/s12967-018-1611-7. 135. Shen, J.; Xiao, Z.; Zhao, Q.; Li, M.; Wu, X.; Zhang, L.; Hu, W.; Cho, C.H. Anti-cancer therapy with TNFα and IFNγ: A comprehensive review. Cell Prolif. 2018, 51, e12441, doi:10.1111/cpr.12441. | pt_BR |
dc.publisher.initials | UEA | pt_BR |
Aparece nas coleções: | DISSERTAÇÃO - PPCAH Programa de Pós-Graduação em Ciências Aplicadas à Hematologia |
Arquivos associados a este item:
Arquivo | Descrição | Tamanho | Formato | |
---|---|---|---|---|
Compostos nanoparticulados - avaliação do potencial antileucêmico e imunomodulador.pdf | 1,92 MB | Adobe PDF | Visualizar/Abrir |
Os itens no repositório estão protegidos por copyright, com todos os direitos reservados, salvo quando é indicado o contrário.