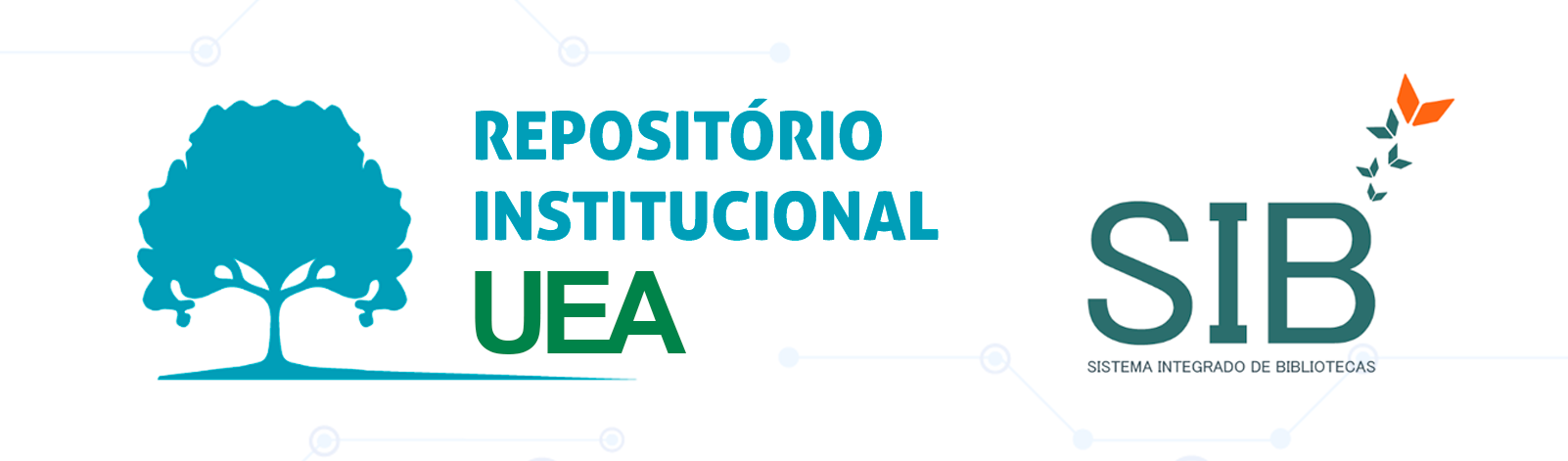
Use este identificador para citar ou linkar para este item:
http://repositorioinstitucional.uea.edu.br//handle/riuea/5342
Registro completo de metadados
Campo DC | Valor | Idioma |
---|---|---|
dc.contributor.author | Soares, Geyse Adriana da Silva | - |
dc.date.available | 2023-11-08 | - |
dc.date.available | 2023-11-21T13:05:33Z | - |
dc.date.issued | 2023-08-21 | - |
dc.identifier.uri | http://repositorioinstitucional.uea.edu.br//handle/riuea/5342 | - |
dc.description.abstract | COVID-19 is an infectious disease of the human respiratory tract caused by SARS-CoV-2. The inflammatory response of COVID-19 is marked by the exacerbated release of pro-inflammatory mediators, causing significant tissue damage. Microvesicles are a type of extracellular vesicle released by all cell types, with functions such as intercellular communication and transporters of biomolecules, being able to induce inflammatory and anti-inflammatory responses. However, little is known about their involvement in the response against SARS-Cov-2 during the acute phase and the convalescent period. Objective: To characterize the profile of subpopulations of circulating microvesicles during the inflammatory process developed in patients infected with SARS-CoV-2 and individuals convalescing from COVID-19. Material and methods: The immunophenotypic characterization of microvesicles, obtained by the flow cytometry technique, was performed in 15 healthy individuals, 9 patients infected with SARS-CoV-2 and 15 convalescing individuals from COVID-19. Results: monocyte (CD14) and platelet (CD41) microvesicles were significantly decreased in patients with COVID-19, as well as in convalescent individuals compared to controls, otherwise there was a significant increase in B lymphocyte microvesicles (CD19) in the patients compared to controls and convalescent subjects. Convalescent individuals showed a higher production of endothelial cell microvesicles (CD51/61) on D30, significantly differing from healthy controls. Conclusion: our study shows that the profile of microvesicles in patients infected with SARS-CoV-2 presents a low release of monocyte and platelet microvesicles, on the other hand, B lymphocyte microvesicles are elevated during the acute phase of the disease. The profile of microvesicles in convalescent individuals showed that endothelial cell microvesicles (CD51/61) were elevated, this shows us that COVID-19, in fact, is a disease that has a very extensive inflammatory response, which causes, even at low degree, a very important tissue damage | pt_BR |
dc.language | por | pt_BR |
dc.publisher | Universidade do Estado do Amazonas | pt_BR |
dc.rights | Acesso Aberto | pt_BR |
dc.subject | SARS-CoV-2 | pt_BR |
dc.subject | COVID-19 | pt_BR |
dc.subject | Microvesículas | pt_BR |
dc.subject | Plasma convalescente | pt_BR |
dc.title | Caracterização do perfil de microvesículas circulantes na infecção por SARS-CoV-2 em pacientes e indivíduos convalescentes | pt_BR |
dc.title.alternative | Characterization of the circulating microvesiculas profile in SARS-CoV-2 infection in patients and convalescent individuals | pt_BR |
dc.type | Dissertação | pt_BR |
dc.date.accessioned | 2023-11-21T13:05:33Z | - |
dc.contributor.advisor-co1 | Tarragô, Andréa Monteiro | - |
dc.contributor.advisor-co1Lattes | http://lattes.cnpq.br/4644326589690231 | pt_BR |
dc.contributor.advisor1 | Marie, Adriana Malheiro Alle | - |
dc.contributor.advisor1Lattes | http://lattes.cnpq.br/2627415957053194 | pt_BR |
dc.contributor.referee1 | Marie, Adriana Malheiro Alle | - |
dc.contributor.referee1Lattes | http://lattes.cnpq.br/2627415957053194 | pt_BR |
dc.contributor.referee2 | Pontes, Letícia Gomes de | - |
dc.contributor.referee2Lattes | http://lattes.cnpq.br/8116355457327399 | pt_BR |
dc.contributor.referee3 | Chaves, Yuri Oliveira | - |
dc.contributor.referee3Lattes | http://lattes.cnpq.br/5379832019495766 | pt_BR |
dc.creator.Lattes | http://lattes.cnpq.br/8722475305347845 | pt_BR |
dc.description.resumo | A COVID-19 é uma doença infecciosa do trato respiratório humano causada pelo SARS-CoV-2. A resposta inflamatória da COVID-19, é marcada pela liberação exacerbada de mediadores pró-inflamatórios, causando danos tecidual importante. Microvesículas são um tipo de vesícula extracelular liberadas por todos os tipos celulares, com funções tais como, de comunicação intercelular e transportadoras de biomoléculas, sendo capazes de induzir respostas inflamatórias e anti-inflamatórias. No entanto, pouco se sabe sobre o envolvimento das mesmas na resposta contra o SARS-Cov-2 durante a fase aguda e no período convalescente. Objetivo: Caracterizar o perfil das subpopulações de microvesículas circulantes durante o processo inflamatório desenvolvido nos pacientes da infecção pelo SARS-CoV-2 e indivíduos convalescente da COVID-19. Material e métodos: A caracterização imunofenotípica das microvesículas, obtida pela técnica de citometria de fluxo, foi realizada em 15 indivíduos saudáveis, 9 pacientes infectados por SARS-CoV-2 e 15 indivíduos convalescentes da COVID-19. Resultados: microvesículas de monócitos (CD14) e de plaquetas (CD41) estavam diminuídas significativamente em pacientes com COVID-19, assim como em indivíduos convalescentes em comparação aos controles, de outro modo houve um aumento significativo das microvesículas de linfócitos B (CD19) nos pacientes em comparação a controles e indivíduos convalescentes. Os indivíduos convalescentes apresentaram uma produção maior de microvesículas de células endoteliais (CD51/61) no D30, diferindo significativamente de controles saudáveis. Conclusão: nosso estudo mostra que o perfil de microvesículas em pacientes infectados com SARS-CoV-2 apresenta uma baixa liberação de microvesículas de monócitos e plaquetas, por outro lado as microvesículas de linfócitos B estão elevadas durante a fase aguda da doença. O perfil de microvesículas em indivíduos convalescentes mostrou que microvesículas de células endoteliais (CD51/61) estavam elevadas, isso nos mostra que a COVID-19, de fato, é uma doença que tem uma resposta inflamatória bastante extensa, que causa, mesmo em baixo grau, um dano tecidual bastante importante. | pt_BR |
dc.publisher.country | Brasil | pt_BR |
dc.publisher.program | PPGH -PROGRAMA DE PÓS-GRADUAÇÃO EM CIÊNCIAS APLICADAS À HEMATOLOGIA | pt_BR |
dc.relation.references | 1. Muralidar S, Ambi SV, Sekaran S, Krishnan UM. The emergence of COVID-19 as a global pandemic: Understanding the epidemiology, immune response and potential therapeutic targets of SARS-CoV-2. Vol. 179, Biochimie. Elsevier B.V.; 2020. p. 85–100. 2. Rothan HA, Byrareddy SN. The epidemiology and pathogenesis of coronavirus disease (COVID-19) outbreak. J Autoimmun [Internet]. 2020 May 1 [cited 2022 Aug 11];109:102433. Available from: /pmc/articles/PMC7127067/ 3. Sahin AR, Erdogan A, Agaoglu PM, Dineri Y, Cakirci AY, Senel ME, et al. 2019 Novel Coronavirus (COVID-19) Outbreak: A Review of the Current Literature. Eurasian J Med Oncol [Internet]. 2020 [cited 2022 Aug 11];4(1):1–7. Available from: https://www.ejmo.org/10.14744/ejmo.2020.12220/ 4. Rabi FA, al Zoubi MS, Al-Nasser AD, Kasasbeh GA, Salameh DM. Sars-cov-2 and coronavirus disease 2019: What we know so far. Vol. 9, Pathogens. MDPI AG; 2020. 5. Kuba K, Imai Y, Rao S, Gao H, Guo F, Guan B, et al. A crucial role of angiotensin converting enzyme 2 (ACE2) in SARS coronavirus–induced lung injury. Nat Med [Internet]. 2005 Aug [cited 2022 Aug 11];11(8):875. Available from: /pmc/articles/PMC7095783/ 6. Heurich A, Hofmann-Winkler H, Gierer S, Liepold T, Jahn O, Pöhlmann S. TMPRSS2 and ADAM17 Cleave ACE2 Differentially and Only Proteolysis by TMPRSS2 Augments Entry Driven by the Severe Acute Respiratory Syndrome Coronavirus Spike Protein. J Virol [Internet]. 2014 Jan 15 [cited 2022 Aug 11];88(2):1293. Available from: /pmc/articles/PMC3911672/ 7. Parasher A. COVID-19: Current understanding of its Pathophysiology, Clinical presentation and Treatment. Vol. 97, Postgraduate Medical Journal. BMJ Publishing Group; 2021. p. 312–20. 8. Tay MZ, Poh CM, Rénia L, MacAry PA, Ng LFP. The trinity of COVID-19: immunity, inflammation and intervention. Nat Rev Immunol [Internet]. 2020 Jun 28;20(6):363–74. Available from: http://www.pubmedcentral.nih.gov/articlerender.fcgi?artid=PMC7187672 9. Harrison AG, Lin T, Wang P. Mechanisms of SARS-CoV-2 Transmission and Pathogenesis. Vol. 41, Trends in Immunology. Elsevier Ltd; 2020. p. 1100–15. 10. Zhou R, To KKW, Wong YC, Liu L, Zhou B, Li X, et al. Acute SARS-CoV-2 Infection Impairs Dendritic Cell and T Cell Responses. Immunity [Internet]. 2020 Oct 10 [cited 2022 Aug 11];53(4):864. Available from: /pmc/articles/PMC7402670/ 11. Yang X, Yu Y, Xu J, Shu H, Xia J, Liu H, et al. Clinical course and outcomes of critically ill patients with SARS-CoV-2 pneumonia in Wuhan, China: a single- 84 centered, retrospective, observational study. Lancet Respir Med [Internet]. 2020 May 1 [cited 2022 Aug 11];8(5):475. Available from: /pmc/articles/PMC7102538/ 12. Wu JT, Leung K, Bushman M, Kishore N, Niehus R, de Salazar PM, et al. Estimating clinical severity of COVID-19 from the transmission dynamics in Wuhan, China. Nat Med [Internet]. 2020 Apr 1 [cited 2022 Aug 11];26(4):506. Available from: /pmc/articles/PMC7094929/ 13. Chen H, Liu W, Liu D, Zhao L, Yu J, Kong H, et al. SARS-CoV-2 activates lung epithelia cell proinflammatory signaling and leads to immune dysregulation in COVID-19 patients by single-cell sequencing. medRxiv [Internet]. 2020 May 13 [cited 2022 Aug 11];2020.05.08.20096024. Available from: https://www.medrxiv.org/content/10.1101/2020.05.08.20096024v1 14. Blanco-Melo D, Nilsson-Payant BE, Liu WC, Uhl S, Hoagland D, Møller R, et al. Imbalanced Host Response to SARS-CoV-2 Drives Development of COVID-19. Cell [Internet]. 2020 May 5 [cited 2022 Aug 11];181(5):1036. Available from: /pmc/articles/PMC7227586/ 15. Qin C, Zhou L, Hu Z, Zhang S, Yang S, Tao Y, et al. Dysregulation of immune response in patients with COVID-19 in Wuhan, China. Clin Infect Dis [Internet]. 2020 Aug 1 [cited 2022 Aug 11];71(15):762–8. Available from: /pmc/articles/PMC7108125/?report=abstract 16. Wang J, Chen S, Bihl J. Exosome-Mediated Transfer of ACE2 (Angiotensin-Converting Enzyme 2) from Endothelial Progenitor Cells Promotes Survival and Function of Endothelial Cell. Oxid Med Cell Longev [Internet]. 2020 [cited 2023 Mar 30];2020. Available from: /pmc/articles/PMC6995312/ 17. Zhu S, Li S, Yi M, Li N, Wu K. Roles of Microvesicles in Tumor Progression and Clinical Applications. Int J Nanomedicine [Internet]. 2021 [cited 2023 Apr 26];16:7071. Available from: /pmc/articles/PMC8536885/ 18. Ratajczak J, Wysoczynski M, Hayek F, Janowska-Wieczorek A, Ratajczak MZ. Membrane-derived microvesicles: important and underappreciated mediators of cell-to-cell communication. Leukemia [Internet]. 2006 [cited 2022 Aug 21];20(9):1487–95. Available from: https://pubmed.ncbi.nlm.nih.gov/16791265/ 19. Hamali HA, Saboor M, Dobie G, Madkhali AM, Akhter MS, Hakamy A, et al. Procoagulant Microvesicles in COVID-19 Patients: Possible Modulators of Inflammation and Prothrombotic Tendency. Infect Drug Resist. 2022;15:2359–68. 20. Andersen KG, Rambaut A, Lipkin WI, Holmes EC, Garry RF. The proximal origin of SARS-CoV-2. Nat Med. 2020;26(April):450–2. 21. Fehr AR, Perlman S. Coronaviruses: An Overview of Their Replication and Pathogenesis. In: Methods in molecular biology (Clifton, NJ) [Internet]. Springer New York; 2015. p. 1–23. Available from: http://link.springer.com/10.1007/978-1-4939-2438-7_1 85 22. Shereen MA, Khan S, Kazmi A, Bashir N, Siddique R. COVID-19 infection: Origin, transmission, and characteristics of human coronaviruses. J Adv Res. 2020 Jul;24:91–8. 23. Weiss SR, Navas-Martin S. Coronavirus pathogenesis and the emerging pathogen severe acute respiratory syndrome coronavirus. Microbiol Mol Biol Rev [Internet]. 2005 Dec;69(4):635–64. Available from: http://www.ncbi.nlm.nih.gov/pubmed/16339739 24. Kitchen LC, Berman M, Halper J, Chazot P. Rationale for 1068 nm Photobiomodulation Therapy (PBMT) as a Novel, Non-Invasive Treatment for COVID-19 and Other Coronaviruses: Roles of NO and Hsp70. International Journal of Molecular Sciences 2022, Vol 23, Page 5221 [Internet]. 2022 May 7 [cited 2023 Apr 11];23(9):5221. Available from: https://www.mdpi.com/1422-0067/23/9/5221/htm 25. Zhang Q, Xiang R, Huo S, Zhou Y, Jiang S, Wang Q, et al. Molecular mechanism of interaction between SARS-CoV-2 and host cells and interventional therapy. Vol. 6, Signal Transduction and Targeted Therapy. Springer Nature; 2021. 26. Muralidar S, Ambi SV, Sekaran S, Krishnan UM. The emergence of COVID-19 as a global pandemic: Understanding the epidemiology, immune response and potential therapeutic targets of SARS-CoV-2. Vol. 179, Biochimie. Elsevier B.V.; 2020. p. 85–100. 27. Rothan HA, Byrareddy SN. The epidemiology and pathogenesis of coronavirus disease (COVID-19) outbreak. J Autoimmun [Internet]. 2020 May 1 [cited 2022 Aug 14];109:102433. Available from: /pmc/articles/PMC7127067/ 28. LaRotta J, Escobar O, Ávila-Aguero ML, Torres JP, Sini de Almeida R, Morales G del C, et al. COVID-19 in Latin America: A Snapshot in Time and the Road Ahead. Infect Dis Ther [Internet]. 2023 Feb 1 [cited 2023 Apr 13];12(2):389–410. Available from: https://link.springer.com/article/10.1007/s40121-022-00748-z 29. de Jesus JG, Sacchi C, Candido D da S, Claro IM, Sales FCS, Manuli ER, et al. Importation and early local transmission of COVID-19 in Brazil, 2020. Rev Inst Med Trop Sao Paulo [Internet]. 2020 [cited 2023 Apr 13];62. Available from: https://pubmed.ncbi.nlm.nih.gov/32401959/ 30. Taylor L. How Latin America is fighting covid-19, for better and worse. BMJ [Internet]. 2020 Sep 1 [cited 2023 Apr 13];370. Available from: https://pubmed.ncbi.nlm.nih.gov/32873574/ 31. Pablos-Méndez A, Vega J, Aranguren FP, Tabish H, Raviglione MC. Covid-19 in Latin America. BMJ [Internet]. 2020 Jul 27 [cited 2023 Apr 13];370. Available from: https://pubmed.ncbi.nlm.nih.gov/32718938/ 86 32. Taylor L. Covid-19: WHO pleads for vaccines as South American countries register record deaths. BMJ [Internet]. 2021 Jun 18 [cited 2023 Apr 13];373:n1587. Available from: https://pubmed.ncbi.nlm.nih.gov/34144947/ 33. Silva SJR da, Pena L. Collapse of the public health system and the emergence of new variants during the second wave of the COVID-19 pandemic in Brazil. One Health [Internet]. 2021 Dec 1 [cited 2023 Apr 13];13:100287. Available from: /pmc/articles/PMC8240439/ 34. Sabino EC, Buss LF, Carvalho MPS, Prete CA, Crispim MAE, Fraiji NA, et al. Resurgence of COVID-19 in Manaus, Brazil, despite high seroprevalence. Lancet [Internet]. 2021 Feb 6 [cited 2023 Apr 13];397(10273):452. Available from: /pmc/articles/PMC7906746/ 35. Barreto IC de HC, Costa Filho RV, Ramos RF, Oliveira LG de, Martins NRAV, Cavalcante FV, et al. Colapso na saúde em Manaus: o fardo de não aderir às medidas não farmacológicas de redução da transmissão da Covid-19. Saúde em Debate [Internet]. 2021 Dec 8 [cited 2023 Apr 16];45(131):1126–39. Available from: http://www.scielo.br/j/sdeb/a/ktbLC8Qcncmt4nKgKgJr6TS/?lang=pt 36. Buss LF, Prete CA, Abrahim CMM, Mendrone A, Salomon T, De Almeida-Neto C, et al. Three-quarters attack rate of SARS-CoV-2 in the Brazilian Amazon during a largely unmitigated epidemic. Science [Internet]. 2021 Jan 15 [cited 2023 Apr 16];371(6526):288–92. Available from: https://pubmed.ncbi.nlm.nih.gov/33293339/ 37. Lumley SF, O’Donnell D, Stoesser NE, Matthews PC, Howarth A, Hatch SB, et al. Antibody Status and Incidence of SARS-CoV-2 Infection in Health Care Workers. New England Journal of Medicine [Internet]. 2021 Feb 11 [cited 2023 Apr 16];384(6):533–40. Available from: https://www.nejm.org/doi/10.1056/NEJMoa2034545 38. Dríade | Dados - Prevalência de coronavírus na Amazônia brasileira e na cidade de São Paulo [Internet]. [cited 2023 Apr 16]. Available from: https://datadryad.org/stash/dataset/doi:10.5061/dryad.c59zw3r5n 39. SARS-CoV-2 reinfection by the new Variant of Concern (VOC) P.1 in Amazonas, Brazil - SARS-CoV-2 coronavirus / nCoV-2019 Genomic Epidemiology - Virological [Internet]. [cited 2023 Apr 16]. Available from: https://virological.org/t/sars-cov-2-reinfection-by-the-new-variant-of-concern-voc-p-1-in-amazonas-brazil/596 40. Genomic characterisation of an emergent SARS-CoV-2 lineage in Manaus: preliminary findings - SARS-CoV-2 coronavirus / nCoV-2019 Genomic Epidemiology - Virological [Internet]. [cited 2023 Apr 16]. Available from: https://virological.org/t/genomic-characterisation-of-an-emergent-sars-cov-2-lineage-in-manaus-preliminary-findings/586 87 41. Zhou Z, Zhu Y, Chu M. Role of COVID-19 Vaccines in SARS-CoV-2 Variants. Front Immunol. 2022 May 20;13. 42. WHO. Tracking SARS-CoV-2 variants [Internet]. [cited 2022 Aug 21]. Available from: https://www.who.int/en/activities/tracking-SARS-CoV-2-variants/ 43. Galloway SE, Paul P, MacCannell DR, Johansson MA, Brooks JT, MacNeil A, et al. Emergence of SARS-CoV-2 B.1.1.7 Lineage — United States, December 29, 2020–January 12, 2021. Morbidity and Mortality Weekly Report [Internet]. 2021 Jan 1 [cited 2022 Aug 21];70(3):95. Available from: /pmc/articles/PMC7821772/ 44. WHO. WHO Coronavirus Disease (COVID-19) Dashboard [Internet]. 2020 [cited 2020 Oct 25]. p. 1. Available from: https://covid19.who.int/ 45. Ministerio da Saúde. Painel Coronavírus [Internet]. 2020 [cited 2020 Oct 25]. p. 1. Available from: https://covid.saude.gov.br/ 46. SUSAM/FVS - Relatório COVID-19 [Internet]. [cited 2022 Sep 3]. Available from: http://www.saude.am.gov.br/painel/corona/ 47. Hartenian E, Nandakumar D, Lari A, Ly M, Tucker JM, Glaunsinger BA. The molecular virology of coronaviruses. Vol. 295, Journal of Biological Chemistry. American Society for Biochemistry and Molecular Biology Inc.; 2020. p. 12910–34. 48. Jiang S, Hillyer C, Du L. Neutralizing Antibodies against SARS-CoV-2 and Other Human Coronaviruses. Trends Immunol [Internet]. 2020 May 1 [cited 2023 Apr 4];41(5):355–9. Available from: http://www.cell.com/article/S1471490620300570/fulltext 49. Guan W jie, Ni Z yi, Hu Y, Liang W hua, Ou C quan, He J xing, et al. Clinical Characteristics of Coronavirus Disease 2019 in China. New England Journal of Medicine. 2020 Apr 30;382(18):1708–20. 50. Li Q, Guan X, Wu P, Wang X, Zhou L, Tong Y, et al. Early Transmission Dynamics in Wuhan, China, of Novel Coronavirus–Infected Pneumonia. New England Journal of Medicine. 2020 Mar 26;382(13):1199–207. 51. Lauer SA, Grantz KH, Bi Q, Jones FK, Zheng Q, Meredith HR, et al. The incubation period of coronavirus disease 2019 (CoVID-19) from publicly reported confirmed cases: Estimation and application. Ann Intern Med. 2020 May 5;172(9):577–82. 52. Yang L, Liu S, Liu J, Zhang Z, Wan X, Huang B, et al. COVID-19: immunopathogenesis and Immunotherapeutics. Signal Transduction and Targeted Therapy 2020 5:1 [Internet]. 2020 Jul 25 [cited 2023 Apr 12];5(1):1–8. Available from: https://www.nature.com/articles/s41392-020-00243-2 53. Huang C, Wang Y, Li X, Ren L, Zhao J, Hu Y, et al. Clinical features of patients infected with 2019 novel coronavirus in Wuhan, China. The Lancet. 2020 Feb 15;395(10223):497–506. 88 54. Chen G, Wu D, Guo W, Cao Y, Huang D, Wang H, et al. Clinical and immunological features of severe and moderate coronavirus disease 2019. Journal of Clinical Investigation. 2020 May 1;130(5):2620–9. 55. Alturki SO, Alturki SO, Connors J, Cusimano G, Kutzler MA, Izmirly AM, et al. The 2020 Pandemic: Current SARS-CoV-2 Vaccine Development. Front Immunol [Internet]. 2020 Aug 19 [cited 2023 Jun 21];11:1880. Available from: /pmc/articles/PMC7466534/ 56. Kucirka LM, Lauer SA, Laeyendecker O, Boon D, Lessler J. Variation in False-Negative Rate of Reverse Transcriptase Polymerase Chain Reaction–Based SARS-CoV-2 Tests by Time Since Exposure. Ann Intern Med [Internet]. 2020 Aug 18 [cited 2022 Aug 31];173(4):262–8. Available from: /pmc/articles/PMC7240870/ 57. Carpenter CR, Mudd PA, West CP, Wilber E, Wilber ST. Diagnosing COVID-19 in the Emergency Department: A Scoping Review of Clinical Examinations, Laboratory Tests, Imaging Accuracy, and Biases. Academic Emergency Medicine [Internet]. 2020 Aug 1 [cited 2022 Aug 31];27(8):653–70. Available from: /pmc/articles/PMC7323136/ 58. Khandker SS, Hashim NHHN, Deris ZZ, Shueb RH, Islam MA. Diagnostic accuracy of rapid antigen test kits for detecting SARS-CoV-2: A systematic review and meta-analysis of 17,171 suspected COVID-19 patients. J Clin Med [Internet]. 2021 Aug 2 [cited 2022 Aug 31];10(16). Available from: /pmc/articles/PMC8397079/ 59. Brümmer LE, Katzenschlager S, Gaeddert M, Erdmann C, Schmitz S, Bota M, et al. Accuracy of novel antigen rapid diagnostics for SARS-CoV-2: A living systematic review and meta-analysis. PLoS Med [Internet]. 2021 Aug 1 [cited 2022 Aug 31];18(8). Available from: /pmc/articles/PMC8389849/ 60. Strömer A, Rose R, Schäfer M, Schön F, Vollersen A, Lorentz T, et al. Performance of a Point-of-Care Test for the Rapid Detection of SARS-CoV-2 Antigen. Microorganisms [Internet]. 2021 Jan 1 [cited 2022 Aug 31];9(1):1–11. Available from: /pmc/articles/PMC7823488/ 61. Long B, Carius BM, Chavez S, Liang SY, Brady WJ, Koyfman A, et al. Clinical update on COVID-19 for the emergency clinician: Presentation and evaluation. Vol. 54, American Journal of Emergency Medicine. W.B. Saunders; 2022. p. 46–57. 62. Li M, Wang H, Tian L, Pang Z, Yang Q, Huang T, et al. COVID-19 vaccine development: milestones, lessons and prospects. Signal Transduct Target Ther [Internet]. 2022 Dec 1 [cited 2023 Apr 12];7(1). Available from: /pmc/articles/PMC9062866/ 63. Zhang H ping, Sun Y li, Wang Y fen, Yazici D, Azkur D, Ogulur I, et al. Recent developments in the immunopathology of COVID-19. Allergy [Internet]. 2023 Feb 89 1 [cited 2023 Apr 12];78(2):369–88. Available from: https://pubmed.ncbi.nlm.nih.gov/36420736/ 64. Wang GL, Wang ZY, Duan LJ, Meng QC, Jiang MD, Cao J, et al. Susceptibility of Circulating SARS-CoV-2 Variants to Neutralization. N Engl J Med [Internet]. 2021 Jun 17 [cited 2023 Apr 12];384(24):2354–6. Available from: /pmc/articles/PMC8063885/ 65. Zhang Y, Wei Y, Yang S, Li Y, Wang J, Nie Z, et al. Rapid and accurate identification of SARS-CoV-2 variants containing E484 mutation. The Innovation [Internet]. 2022 Jan 1 [cited 2023 Apr 12];3(1):100183. Available from: /pmc/articles/PMC8577878/ 66. Goel RR, Apostolidis SA, Painter MM, Mathew D, Pattekar A, Kuthuru O, et al. Distinct antibody and memory B cell responses in SARS-CoV-2 naïve and recovered individuals following mRNA vaccination. Sci Immunol [Internet]. 2021 Apr 4 [cited 2023 Apr 12];6(58):1–19. Available from: /pmc/articles/PMC8158969/ 67. Duan K, Liu B, Li C, Zhang H, Yu T, Qu J, et al. Effectiveness of convalescent plasma therapy in severe COVID-19 patients. Proc Natl Acad Sci U S A [Internet]. 2020 Apr 28 [cited 2023 Apr 24];117(17):9490–6. Available from: /pmc/articles/PMC7196837/ 68. Shen C, Wang Z, Zhao F, Yang Y, Li J, Yuan J, et al. Treatment of 5 Critically Ill Patients With COVID-19 With Convalescent Plasma. JAMA [Internet]. 2020 Apr 4 [cited 2023 Apr 24];323(16):1582. Available from: /pmc/articles/PMC7101507/ 69. Hu B, Guo H, Zhou P, Shi ZL. Characteristics of SARS-CoV-2 and COVID-19. Nat Rev Microbiol [Internet]. 2021 Mar 1 [cited 2023 Apr 24];19(3):141. Available from: /pmc/articles/PMC7537588/ 70. Rimmer A. Covid-19: Impact of long term symptoms will be profound, warns BMA. BMJ [Internet]. 2020 Aug 13 [cited 2023 Apr 24];370:m3218. Available from: https://pubmed.ncbi.nlm.nih.gov/32816748/ 71. Greenhalgh T, Knight M, A’Court C, Buxton M, Husain L. Management of post-acute covid-19 in primary care. BMJ [Internet]. 2020 Aug 11 [cited 2023 Apr 24];370. Available from: https://pubmed.ncbi.nlm.nih.gov/32784198/ 72. Tarazona-Fernández A, Rauch-Sánchez E, Herrera-Alania O, Galán-Rodas E, Tarazona-Fernández A, Rauch-Sánchez E, et al. ¿Enfermedad prolongada o secuela pos-COVID-19? Acta Médica Peruana [Internet]. 2020 [cited 2023 Apr 24];37(4):565–70. Available from: http://www.scielo.org.pe/scielo.php?script=sci_arttext&pid=S1728-59172020000400565&lng=es&nrm=iso&tlng=es 73. Marsán-Suárez V, Casado-Hernández I, Hernández-Ramos E, Díaz-Domínguez G, Triana-Marrero Y, Duarte-Pérez Y, et al. Biomarkers of sequela in adult patients 90 convalescing from COVID-19. Adv Biomark Sci Technol [Internet]. 2022 [cited 2023 Apr 25];4:36. Available from: /pmc/articles/PMC9645947/ 74. Kang CK, Kim M, Hong J, Kim G, Lee S, Chang E, et al. Distinct Immune Response at 1 Year Post-COVID-19 According to Disease Severity. Front Immunol [Internet]. 2022 Mar 21 [cited 2023 Apr 25];13:1. Available from: /pmc/articles/PMC8980227/ 75. Mehandru S, Merad M. Pathological sequelae of long-haul COVID. Nat Immunol [Internet]. 2022 Feb 1 [cited 2023 Apr 25];23(2):194. Available from: /pmc/articles/PMC9127978/ 76. Phetsouphanh C, Darley DR, Wilson DB, Howe A, Munier CML, Patel SK, et al. Immunological dysfunction persists for 8 months following initial mild-to-moderate SARS-CoV-2 infection. Nat Immunol [Internet]. 2022 Feb 1 [cited 2023 Apr 25];23(2):210–6. Available from: https://pubmed.ncbi.nlm.nih.gov/35027728/ 77. Xu Z, Shi L, Wang Y, Zhang J, Huang L, Zhang C, et al. Pathological findings of COVID-19 associated with acute respiratory distress syndrome. Lancet Respir Med. 2020 Apr;8(4):420–2. 78. Zhang B, Zhou X, Qiu Y, Song Y, Feng F, Feng J, et al. Clinical characteristics of 82 cases of death from COVID-19. Jin X, editor. PLoS One. 2020 Jul 9;15(7):e0235458. 79. Wong CK, Lam CWK, Wu AKL, Ip WK, Lee NLS, Chan IHS, et al. Plasma inflammatory cytokines and chemokines in severe acute respiratory syndrome. Clin Exp Immunol. 2004 Apr;136(1):95–103. 80. Li T, Qiu Z, Zhang L, Han Y, He W, Liu Z, et al. Significant changes of peripheral T lymphocyte subsets in patients with severe acute respiratory syndrome. J Infect Dis [Internet]. 2004 Feb 15 [cited 2023 Apr 12];189(4):648–51. Available from: https://pubmed.ncbi.nlm.nih.gov/14767818/ 81. Tan M, Liu Y, Zhou R, Deng X, Li F, Liang K, et al. Immunopathological characteristics of coronavirus disease 2019 cases in Guangzhou, China. Immunology [Internet]. 2020 Jul 1 [cited 2023 Apr 12];160(3):261. Available from: /pmc/articles/PMC7283723/ 82. Qin C, Zhou L, Hu Z, Zhang S, Yang S, Tao Y, et al. Dysregulation of Immune Response in Patients With Coronavirus 2019 (COVID-19) in Wuhan, China. Clinical Infectious Diseases [Internet]. 2020 Jul 28 [cited 2023 Apr 12];71(15):762–8. Available from: https://academic.oup.com/cid/article/71/15/762/5803306 83. Zhang B, Zhou X, Zhu C, Feng F, Qiu Y, Feng J, et al. Immune phenotyping based on neutrophil-to-lymphocyte ratio and IgG predicts disease severity and outcome for patients with COVID-19. medRxiv [Internet]. 2020 Mar 16 [cited 2023 Apr 12];2020.03.12.20035048. Available from: https://www.medrxiv.org/content/10.1101/2020.03.12.20035048v1 91 84. Liu Y, Sun W, Li J, Chen L, Wang Y, Zhang L, et al. Clinical features and progression of acute respiratory distress syndrome in coronavirus disease 2019. medRxiv [Internet]. 2020 Feb 27 [cited 2023 Apr 12];2020.02.17.20024166. Available from: https://www.medrxiv.org/content/10.1101/2020.02.17.20024166v3 85. Barberis E, Vanella V V., Falasca M, Caneapero V, Cappellano G, Raineri D, et al. Circulating Exosomes Are Strongly Involved in SARS-CoV-2 Infection. Front Mol Biosci. 2021 Feb 22;8:29. 86. Mao K, Tan Q, Ma Y, Wang S, Zhong H, Liao Y, et al. Proteomics of extracellular vesicles in plasma reveals the characteristics and residual traces of COVID-19 patients without underlying diseases after 3 months of recovery. Cell Death & Disease 2021 12:6 [Internet]. 2021 May 25 [cited 2023 Apr 12];12(6):1–18. Available from: https://www.nature.com/articles/s41419-021-03816-3 87. Moraes EC dos S, Martins-Gonçalves R, Silva LR da, Mandacaru SC, Melo RM, Azevedo-Quintanilha I, et al. Proteomic Profile of Procoagulant Extracellular Vesicles Reflects Complement System Activation and Platelet Hyperreactivity of Patients with Severe COVID-19. Front Cell Infect Microbiol. 2022 Jul 22;12:981. 88. Maugeri N, De Lorenzo R, Clementi N, Antonia Diotti R, Criscuolo E, Godino C, et al. Unconventional CD147‐dependent platelet activation elicited by SARS‐CoV‐2 in COVID‐19. Journal of Thrombosis and Haemostasis [Internet]. 2022 Feb 1 [cited 2023 Apr 10];20(2):434. Available from: /pmc/articles/PMC8646617/ 89. Manfredi AA, Ramirez GA, Godino C, Capobianco A, Monno A, Franchini S, et al. Platelet Phagocytosis via P-selectin Glycoprotein Ligand 1 and Accumulation of Microparticles in Systemic Sclerosis. Arthritis Rheumatol [Internet]. 2022 Feb 1 [cited 2023 Apr 10];74(2):318–28. Available from: https://pubmed.ncbi.nlm.nih.gov/34279048/ 90. Maugeri N, Capobianco A, Rovere-Querini P, Ramirez GA, Tombetti E, Della Valle P, et al. Platelet microparticles sustain autophagy-associated activation of neutrophils in systemic sclerosis. Sci Transl Med [Internet]. 2018 Jul 25 [cited 2023 Apr 10];10(451). Available from: https://pubmed.ncbi.nlm.nih.gov/30045975/ 91. Krishnamachary B, Cook C, Spikes L, Chalise P, Dhillon NK. The Potential Role of Extracellular Vesicles in COVID-19 Associated Endothelial injury and Pro-inflammation. medRxiv [Internet]. 2020 Sep 1; Available from: http://www.ncbi.nlm.nih.gov/pubmed/32909001 92. Kowal J, Tkach M, Théry C, Kowal J, Tkach M, Biogenesis CT. Biogenesis and secretion of exosomes. elsevier. 2020;116–25. 93. Gurunathan S, Kang MH, Qasim M, Khan K, Kim JH. Biogenesis, membrane trafficking, functions, and next generation nanotherapeutics medicine of extracellular vesicles. Int J Nanomedicine. 2021;16:3357–83. 92 94. Théry C, Witwer KW, Aikawa E, Alcaraz MJ, Anderson JD, Andriantsitohaina R, et al. Minimal information for studies of extracellular vesicles 2018 (MISEV2018): a position statement of the International Society for Extracellular Vesicles and update of the MISEV2014 guidelines. J Extracell Vesicles. 2018 Dec 1;7(1):1535750. 95. Witwer KW, Théry C. Extracellular vesicles or exosomes? On primacy, precision, and popularity influencing a choice of nomenclature. Vol. 8, Journal of Extracellular Vesicles. Taylor and Francis Ltd.; 2019. 96. Chen Y, Li G, Liu ML. Microvesicles as Emerging Biomarkers and Therapeutic Targets in Cardiometabolic Diseases. Vol. 16, Genomics, Proteomics and Bioinformatics. Beijing Genomics Institute; 2018. p. 50–62. 97. van Niel G, Charrin S, Simoes S, Romao M, Rochin L, Saftig P, et al. The tetraspanin CD63 regulates ESCRT-independent and dependent endosomal sorting during melanogenesis. Dev Cell [Internet]. 2011 Oct 10 [cited 2022 Jul 17];21(4):708. Available from: /pmc/articles/PMC3199340/ 98. Hsu C, Morohashi Y, Yoshimura SI, Manrique-Hoyos N, Jung SY, Lauterbach MA, et al. Regulation of exosome secretion by Rab35 and its GTPase-activating proteins TBC1D10A-C. Journal of Cell Biology. 2010 Apr 19;189(2):223–32. 99. Ståhl A lie, Johansson K, Mossberg M, Kahn R, Karpman D. Exosomes and microvesicles in normal physiology, pathophysiology, and renal diseases. Vol. 34, Pediatric Nephrology. Springer Verlag; 2019. p. 11–30. 100. Yáñez-Mó M, Siljander PRM, Andreu Z, Zavec AB, Borràs FE, Buzas EI, et al. Biological properties of extracellular vesicles and their physiological functions. J Extracell Vesicles [Internet]. 2015 [cited 2022 Jul 20];4(2015):1–60. Available from: /pmc/articles/PMC4433489/ 101. Dragovic RA, Gardiner C, Brooks AS, Tannetta DS, Ferguson DJP, Hole P, et al. Sizing and phenotyping of cellular vesicles using Nanoparticle Tracking Analysis. Nanomedicine [Internet]. 2011 Dec [cited 2022 Jul 20];7(6):780. Available from: /pmc/articles/PMC3280380/ 102. van der Pol E, Coumans FAW, Grootemaat AE, Gardiner C, Sargent IL, Harrison P, et al. Particle size distribution of exosomes and microvesicles determined by transmission electron microscopy, flow cytometry, nanoparticle tracking analysis, and resistive pulse sensing. J Thromb Haemost [Internet]. 2014 [cited 2022 Jul 20];12(7):1182–92. Available from: https://pubmed.ncbi.nlm.nih.gov/24818656/ 103. Ståhl A lie, Johansson K, Mossberg M, Kahn R, Karpman D. Exosomes and microvesicles in normal physiology, pathophysiology, and renal diseases Anne-lie. Pediatric Nephrology. 2019;11–30. 104. Segura E, Amigorena S, Théry C. Mature dendritic cells secrete exosomes with strong ability to induce antigen-specific effector immune responses. Blood Cells 93 Mol Dis [Internet]. 2005 Sep [cited 2022 Jul 20];35(2):89–93. Available from: https://pubmed.ncbi.nlm.nih.gov/15990342/ 105. Obregon C, Rothen-Rutishauser B, Gerber P, Gehr P, Nicod LP. Active Uptake of Dendritic Cell-Derived Exovesicles by Epithelial Cells Induces the Release of Inflammatory Mediators through a TNF-α-Mediated Pathway. Am J Pathol [Internet]. 2009 [cited 2022 Jul 20];175(2):696. Available from: /pmc/articles/PMC2715287/ 106. Munich S, Sobo-Vujanovic A, Buchser WJ, Beer-Stolz D, Vujanovic NL. Dendritic cell exosomes directly kill tumor cells and activate natural killer cells via TNF superfamily ligands. Oncoimmunology [Internet]. 2012 Oct 10 [cited 2022 Jul 20];1(7):1074. Available from: /pmc/articles/PMC3494621/ 107. Vanni I, Alama A, Grossi F, Dal Bello MG, Coco S. Exosomes: a new horizon in lung cancer. Drug Discov Today. 2017 Jun 1;22(6):927–36. 108. Kadota T, Yoshioka Y, Fujita Y, Kuwano K, Ochiya T. Extracellular vesicles in lung cancer—From bench to bedside. Semin Cell Dev Biol. 2017 Jul 1;67:39–47. 109. Mesri M, Altieri DC. Leukocyte Microparticles Stimulate Endothelial Cell Cytokine Release and Tissue Factor Induction in a JNK1 Signaling Pathway* [Internet]. 1999. Available from: http://www.jbc.org/ 110. Distler JHW, Huber LC, Gay S, Distler O, Pisetsky DS. Microparticles as mediators of cellular cross-talk in inflammatory disease. Autoimmunity [Internet]. 2006 Dec [cited 2022 Jul 20];39(8):683–90. Available from: https://pubmed.ncbi.nlm.nih.gov/17178565/ 111. Gasser O, Schifferli JA. Activated polymorphonuclear neutrophils disseminate anti-inflammatory microparticles by ectocytosis. Blood [Internet]. 2004 Oct 15 [cited 2022 Jul 20];104(8):2543–8. Available from: https://pubmed.ncbi.nlm.nih.gov/15213101/ 112. Mesri M, Altieri DC. Microparticles Endothelial Cell Activation by Leukocyte [Internet]. Vol. 4382, J Immunol References. 1998. Available from: http://www.jimmunol.org/content/161/8/http://www.jimmunol.org/content/161/8/4382.full#ref-list-1 113. del Conde I, Shrimpton CN, Thiagarajan P, López JA. Tissue-factor-bearing microvesicles arise from lipid rafts and fuse with activated platelets to initiate coagulation. Blood [Internet]. 2005 Sep 1 [cited 2022 Jul 20];106(5):1604–11. Available from: https://pubmed.ncbi.nlm.nih.gov/15741221/ 114. Hartwig JH, Chambers KA, Stossel TP. Association of gelsolin with actin filaments and cell membranes of macrophages and platelets. J Cell Biol [Internet]. 1989 Feb 2 [cited 2022 Jul 20];108(2):467. Available from: /pmc/articles/PMC2115434/?report=abstract 94 115. Pasquet JM, Dachary-Prigent J, Nurden AT. Calcium influx is a determining factor of calpain activation and microparticle formation in platelets. Eur J Biochem [Internet]. 1996 [cited 2022 Jul 20];239(3):647–54. Available from: https://pubmed.ncbi.nlm.nih.gov/8774708/ 116. VanWijk MJ, VanBavel E, Sturk A, Nieuwland R. Microparticles in cardiovascular diseases. Cardiovasc Res [Internet]. 2003 Aug 1 [cited 2022 Jul 20];59(2):277–87. Available from: https://pubmed.ncbi.nlm.nih.gov/12909311/ 117. Hugel B, Martínez MC, Kunzelmann C, Freyssinet JM. Membrane microparticles: two sides of the coin. Physiology (Bethesda) [Internet]. 2005 [cited 2022 Jul 20];20(1):22–7. Available from: https://pubmed.ncbi.nlm.nih.gov/15653836/ 118. Ratajczak J, Wysoczynski M, Hayek F, Janowska-Wieczorek A, Ratajczak MZ. Membrane-derived microvesicles: important and underappreciated mediators of cell-to-cell communication. Leukemia [Internet]. 2006 [cited 2022 Jul 20];20(9):1487–95. Available from: https://pubmed.ncbi.nlm.nih.gov/16791265/ 119. Connor DE, Exner T, Ma DDF, Joseph JE. The majority of circulating platelet-derived microparticles fail to bind annexin V, lack phospholipid-dependent procoagulant activity and demonstrate greater expression of glycoprotein Ib. Thromb Haemost [Internet]. 2010 [cited 2022 Jul 21];103(5):1044–52. Available from: https://pubmed.ncbi.nlm.nih.gov/20390225/ 120. Turturici G, Tinnirello R, Sconzo G, Geraci F. Extracellular membrane vesicles as a mechanism of cell-to-cell communication: advantages and disadvantages. Am J Physiol Cell Physiol [Internet]. 2014 Apr 1 [cited 2022 Jul 21];306(7). Available from: https://pubmed.ncbi.nlm.nih.gov/24452373/ 121. Surman M, Stępień E, Hoja-Łukowicz D, Przybyło M. Deciphering the role of ectosomes in cancer development and progression: focus on the proteome. Clin Exp Metastasis [Internet]. 2017 Apr 1 [cited 2023 Apr 27];34(3–4):273–89. Available from: https://pubmed.ncbi.nlm.nih.gov/28317069/ 122. Ratajczak MZ, Ratajczak J. Extracellular microvesicles/exosomes: discovery, disbelief, acceptance, and the future? Vol. 34, Leukemia. Springer Nature; 2020. p. 3126–35. 123. Dow R, Ridger V. Neutrophil microvesicles and their role in disease. International Journal of Biochemistry and Cell Biology. 2021 Dec 1;141. 124. Kolonics F, Kajdácsi E, Farkas VJ, Veres DS, Khamari D, Kittel Á, et al. Neutrophils produce proinflammatory or anti-inflammatory extracellular vesicles depending on the environmental conditions. J Leukoc Biol [Internet]. 2021 Apr 1 [cited 2022 Aug 21];109(4):793–806. Available from: https://onlinelibrary.wiley.com/doi/full/10.1002/JLB.3A0320-210R 125. Bonaventura A, Montecucco F, Dallegri F, Carbone F, Lüscher TF, Camici GG, et al. Novel findings in neutrophil biology and their impact on cardiovascular disease. 95 Cardiovasc Res [Internet]. 2019 Jul 1 [cited 2023 Apr 16];115(8):1266–85. Available from: https://academic.oup.com/cardiovascres/article/115/8/1266/5421166 126. Soni S, Garner JL, O’Dea KP, Koh M, Finney L, Tirlapur N, et al. Intra-alveolar neutrophil-derived microvesicles are associated with disease severity in COPD. Am J Physiol Lung Cell Mol Physiol [Internet]. 2021 [cited 2023 Apr 17];320(1):L73–83. Available from: https://pubmed.ncbi.nlm.nih.gov/33146567/ 127. Pitanga TN, de Aragão França L, Rocha VCJ, Meirelles T, Matos Borges V, Gonçalves MS, et al. Neutrophil-derived microparticles induce myeloperoxidase-mediated damage of vascular endothelial cells. BMC Cell Biol [Internet]. 2014 Jun 11 [cited 2023 Apr 17];15(1). Available from: https://pubmed.ncbi.nlm.nih.gov/24915973/ 128. Dengler V, Downey GP, Tuder RM, Eltzschig HK, Schmidt EP. Neutrophil intercellular communication in acute lung injury. Emerging roles of microparticles and gap junctions. Am J Respir Cell Mol Biol [Internet]. 2013 Jul [cited 2023 Apr 17];49(1):1–5. Available from: https://pubmed.ncbi.nlm.nih.gov/23815257/ 129. Rhys HI, Dell’Accio F, Pitzalis C, Moore A, Norling L V., Perretti M. Neutrophil Microvesicles from Healthy Control and Rheumatoid Arthritis Patients Prevent the Inflammatory Activation of Macrophages. EBioMedicine [Internet]. 2018 Mar 1 [cited 2023 Apr 17];29:60–9. Available from: https://pubmed.ncbi.nlm.nih.gov/29449195/ 130. Jansen F, Yang X, Franklin BS, Hoelscher M, Schmitz T, Bedorf J, et al. High glucose condition increases NADPH oxidase activity in endothelial microparticles that promote vascular inflammation. Cardiovasc Res [Internet]. 2013 Apr 1 [cited 2022 Aug 21];98(1):94–106. Available from: https://academic.oup.com/cardiovascres/article/98/1/94/313999 131. Vítková V, Živný J, Janota J. Endothelial cell-derived microvesicles: Potential mediators and biomarkers of pathologic processes. Vol. 12, Biomarkers in Medicine. Future Medicine Ltd.; 2018. p. 161–75. 132. Kowal J, Tkach M. Dendritic cell extracellular vesicles. Int Rev Cell Mol Biol [Internet]. 2019 Jan 1 [cited 2023 Apr 19];349:213–49. Available from: https://pubmed.ncbi.nlm.nih.gov/31759432/ 133. Angelot F, Seillès E, Biichlé S, Berda Y, Gaugler B, Plumas J, et al. Endothelial cell-derived microparticles induce plasmacytoid dendritic cell maturation: potential implications in inflammatory diseases. Haematologica [Internet]. 2009 Nov [cited 2023 Apr 19];94(11):1502–12. Available from: https://pubmed.ncbi.nlm.nih.gov/19648164/ 96 134. Combes V, Simon AC, Grau GE, Arnoux D, Camoin L, Sabatier F, et al. In vitro generation of endothelial microparticles and possible prothrombotic activity in patients with lupus anticoagulant. J Clin Invest. 1999 Jul 1;104(1):93–102. 135. Gkaliagkousi E, Nikolaidou B, Gavriilaki E, Lazaridis A, Yiannaki E, Anyfanti P, et al. Increased erythrocyte- and platelet-derived microvesicles in newly diagnosed type 2 diabetes mellitus. Diab Vasc Dis Res. 2019 Sep 1;16(5):458–65. 136. Ye W, Chew M, Hou J, Lai F, Leopold SJ, Loo HL, et al. Microvesicles from malaria-infected red blood cells activate natural killer cells via MDA5 pathway. PLoS Pathog [Internet]. 2018 Oct 1 [cited 2023 Apr 19];14(10). Available from: https://pubmed.ncbi.nlm.nih.gov/30286211/ 137. Ye W, Chew M, Hou J, Lai F, Leopold SJ, Loo HL, et al. Microvesicles from malaria-infected red blood cells activate natural killer cells via MDA5 pathway. PLoS Pathog [Internet]. 2018 Oct 1 [cited 2023 Apr 19];14(10). Available from: https://pubmed.ncbi.nlm.nih.gov/30286211/ 138. Stavrou EX. Thromboinflammatory effects of RBC microvesicles. Blood [Internet]. 2020 Mar 5 [cited 2023 Apr 19];135(10):708–9. Available from: https://pubmed.ncbi.nlm.nih.gov/32135018/ 139. Strack K, Lauri N, Maté SM, Saralegui A, Muñoz-Garay C, Schwarzbaum PJ, et al. Induction of erythrocyte microvesicles by Escherichia Coli Alpha hemolysin. Biochem J [Internet]. 2019 [cited 2023 Apr 19];476(22):3455–73. Available from: https://pubmed.ncbi.nlm.nih.gov/31661116/ 140. Noubouossie DF, Henderson MW, Mooberry M, Ilich A, Ellsworth P, Piegore M, et al. Red blood cell microvesicles activate the contact system, leading to factor IX activation via 2 independent pathways. Blood [Internet]. 2020 Mar 5 [cited 2023 Apr 19];135(10):755–65. Available from: https://ashpublications.org/blood/article/135/10/755/431281/Red-blood-cell-microvesicles-activate-the-contact 141. Shefler I, Salamon P, Reshef T, Mor A, Mekori YA. T cell-induced mast cell activation: a role for microparticles released from activated T cells. J Immunol [Internet]. 2010 Oct 1 [cited 2022 Aug 21];185(7):4206–12. Available from: https://pubmed.ncbi.nlm.nih.gov/20810987/ 142. Shefler I, Pasmanik-Chor M, Kidron D, Mekori YA, Hershko AY. T cell-derived microvesicles induce mast cell production of IL-24: Relevance to inflammatory skin diseases. Journal of Allergy and Clinical Immunology. 2014;133(1). 143. Kornek M, Popov Y, Libermann TA, Afdhal NH, Schuppan D. Human T cell microparticles circulate in blood of hepatitis patients and induce fibrolytic activation of hepatic stellate cells. Hepatology [Internet]. 2011 Jan [cited 2023 Apr 19];53(1):230–42. Available from: https://pubmed.ncbi.nlm.nih.gov/20979056/ 97 144. Vdovenko D, Balbi C, Di Silvestre D, Passignani G, Puspitasari YM, Zarak-Crnkovic M, et al. Microvesicles released from activated CD4+ T cells alter microvascular endothelial cell function. Eur J Clin Invest [Internet]. 2022 Jun 1 [cited 2023 Apr 19];52(6). Available from: https://pubmed.ncbi.nlm.nih.gov/35316536/ 145. Ghosh AK, Secreto CR, Knox TR, Ding W, Mukhopadhyay D, Kay NE. Circulating microvesicles in B-cell chronic lymphocytic leukemia can stimulate marrow stromal cells: implications for disease progression. Blood [Internet]. 2010 Mar 4 [cited 2023 Apr 23];115(9):1755–64. Available from: https://pubmed.ncbi.nlm.nih.gov/20018914/ 146. Ayre DC, Chute IC, Joy AP, Barnett DA, Hogan AM, Grüll MP, et al. CD24 induces changes to the surface receptors of B cell microvesicles with variable effects on their RNA and protein cargo. Sci Rep [Internet]. 2017 Dec 1 [cited 2023 Apr 23];7(1). Available from: https://pubmed.ncbi.nlm.nih.gov/28819186/ 147. Miguet L, Béchade G, Fornecker L, Zink E, Felden C, Gervais C, et al. Proteomic analysis of malignant B-cell derived microparticles reveals CD148 as a potentially useful antigenic biomarker for mantle cell lymphoma diagnosis. J Proteome Res [Internet]. 2009 Jul 6 [cited 2023 Apr 23];8(7):3346–54. Available from: https://pubmed.ncbi.nlm.nih.gov/19413345/ 148. Goubran H, Sabry W, Kotb R, Seghatchian J, Burnouf T. Platelet microparticles and cancer: An intimate cross-talk. Transfus Apher Sci [Internet]. 2015 Oct 1 [cited 2022 Aug 21];53(2):168–72. Available from: https://pubmed.ncbi.nlm.nih.gov/26542350/ 149. Lazar S, Goldfinger LE. Platelets and extracellular vesicles and their cross talk with cancer. Blood [Internet]. 2021 Jun 10;137(23):3192–200. Available from: https://ashpublications.org/blood/article/137/23/3192/475897/Platelets-and-extracellular-vesicles-and-their 150. Yan J, Bao H, Fan YJ, Jiang ZL, Qi YX, Han Y. Platelet-derived microvesicles promote endothelial progenitor cell proliferation in intimal injury by delivering TGF-β1. FEBS J [Internet]. 2020 Dec 1 [cited 2023 Apr 19];287(23):5196–217. Available from: https://onlinelibrary.wiley.com/doi/full/10.1111/febs.15293 151. Suades R, Padró T, Vilahur G, Badimon L. Platelet-released extracellular vesicles: the effects of thrombin activation. Cell Mol Life Sci [Internet]. 2022 Mar 1 [cited 2023 Apr 19];79(3). Available from: https://pubmed.ncbi.nlm.nih.gov/35288766/ 152. Rautou PE, Leroyer AS, Ramkhelawon B, Devue C, Duflaut D, Vion AC, et al. Microparticles from human atherosclerotic plaques promote endothelial ICAM-1-dependent monocyte adhesion and transendothelial migration. Circ Res [Internet]. 2011 Feb 4 [cited 2022 Aug 21];108(3):335–43. Available from: https://pubmed.ncbi.nlm.nih.gov/21164106/ 98 153. Wang JG, Williams JC, Davis BK, Jacobson K, Doerschuk CM, Ting JPY, et al. Monocytic microparticles activate endothelial cells in an IL-1β–dependent manner. Blood [Internet]. 2011 Aug 8 [cited 2022 Aug 21];118(8):2366. Available from: /pmc/articles/PMC3162361/ 154. Brambilla M, Talmon M, Canzano P, Fresu LG, Brunelleschi S, Tremoli E, et al. Different Contribution of Monocyte-and Platelet-Derived Microvesicles to Endothelial Behavior. Int J Mol Sci. 2022 May 1;23(9). 155. Chung SY, Tong MS, Sheu JJ, Lee FY, Sung PH, Chen CJ, et al. Short-term and long-term prognostic outcomes of patients with ST-segment elevation myocardial infarction complicated by profound cardiogenic shock undergoing early extracorporeal membrane oxygenator-assisted primary percutaneous coronary intervention. Int J Cardiol [Internet]. 2016 Nov 15 [cited 2023 Apr 23];223:412–7. Available from: https://pubmed.ncbi.nlm.nih.gov/27544596/ 156. Nomura S, Ozaki Y, Ikeda Y. Function and role of microparticles in various clinical settings. Thromb Res [Internet]. 2008 [cited 2023 Apr 23];123(1):8–23. Available from: https://pubmed.ncbi.nlm.nih.gov/18667228/ 157. Steppich BA, Braun SL, Stein A, Demetz G, Groha P, Schömig A, et al. Plasma TF activity predicts cardiovascular mortality in patients with acute myocardial infarction. Thromb J [Internet]. 2009 Jul 2 [cited 2023 Apr 23];7:11. Available from: /pmc/articles/PMC2714503/ 158. Chiva-Blanch G, Laake K, Myhre P, Bratseth V, Arnesen H, Solheim S, et al. Platelet-, monocyte-derived and tissue factor-carrying circulating microparticles are related to acute myocardial infarction severity. PLoS One [Internet]. 2017 Feb 1 [cited 2023 Apr 23];12(2). Available from: /pmc/articles/PMC5313202/ 159. Guervilly C, Bonifay A, Burtey S, Sabatier F, Cauchois R, Abdili E, et al. Dissemination of extreme levels of extracellular vesicles: tissue factor activity in patients with severe COVID-19. Blood Adv [Internet]. 2021 Feb 2 [cited 2022 Aug 24];5(3):628. Available from: /pmc/articles/PMC7846479/ 160. Nieri D, Neri T, Petrini S, Vagaggini B, Paggiaro P, Celi A. Cell-derived microparticles and the lung. Eur Respir Rev [Internet]. 2016 Sep 1 [cited 2022 Aug 24];25(141):266–77. Available from: https://pubmed.ncbi.nlm.nih.gov/27581826/ 161. Sinauridze EI, Kireev DA, Popenko NY, Pichugin A v., Panteleev MA, Krymskaya O v., et al. Platelet microparticle membranes have 50- to 100-fold higher specific procoagulant activity than activated platelets. Thromb Haemost [Internet]. 2007 Mar [cited 2022 Aug 24];97(3):425–34. Available from: http://www.thieme-connect.de/products/ejournals/html/10.1160/TH06-06-0313 162. Garnier Y, Claude L, Hermand P, Sachou E, Claes A, Desplan K, et al. Plasma microparticles of intubated COVID-19 patients cause endothelial cell death, neutrophil adhesion and netosis, in a phosphatidylserine-dependent manner. Br J 99 Haematol [Internet]. 2022 Mar 1 [cited 2023 Apr 19];196(5):1159–69. Available from: https://onlinelibrary.wiley.com/doi/full/10.1111/bjh.18019 163. Taylor R. Interpretation of the Correlation Coefficient: A Basic Review. Journal of Diagnostic Medical Sonography. 1990;6(1):35–9. 164. Buzas EI. The roles of extracellular vesicles in the immune system. Nature Reviews Immunology. Nature Research; 2022. 165. Tőkés-Füzesi M, Ruzsics I, Rideg O, Kustán P, Kovács GL, Molnár T. Role of microparticles derived from monocytes, endothelial cells and platelets in the exacerbation of COPD. International Journal of COPD. 2018;13:3749–57. 166. Mohan A, Agarwal S, Clauss M, Britt NS, Dhillon NK. Extracellular vesicles: Novel communicators in lung diseases. Vol. 21, Respiratory Research. BioMed Central; 2020. 167. Rand ML, Wang H, Bang KWA, Packham MA, Freedman J. Rapid clearance of procoagulant platelet-derived microparticles from the circulation of rabbits. J Thromb Haemost [Internet]. 2006 Jul [cited 2023 Jul 2];4(7):1621–3. Available from: https://pubmed.ncbi.nlm.nih.gov/16839364/ 168. Yáñez-Mó M, Siljander PRM, Andreu Z, Zavec AB, Borràs FE, Buzas EI, et al. Biological properties of extracellular vesicles and their physiological functions. J Extracell Vesicles [Internet]. 2015 [cited 2023 Jul 2];4(2015):1–60. Available from: /pmc/articles/PMC4433489/ 169. Willekens FLA, Werre JM, Kruijt JK, Roerdinkholder-Stoelwinder B, Groenen-Döpp YAM, Van Den Bos AG, et al. Liver Kupffer cells rapidly remove red blood cell-derived vesicles from the circulation by scavenger receptors. Blood [Internet]. 2005 Mar 1 [cited 2023 Jul 2];105(5):2141–5. Available from: https://pubmed.ncbi.nlm.nih.gov/15550489/ 170. Zahran AM, El-Badawy O, Ali WA, Mahran ZG, Mahran EEMO, Rayan A. Circulating microparticles and activated platelets as novel prognostic biomarkers in COVID-19; relation to cancer. PLoS One [Internet]. 2021 Feb 1 [cited 2023 Jun 6];16(2). Available from: /pmc/articles/PMC7899358/ 171. Puga ML, Menegueti MG, Silvestrini MMA, de Souza Santos LJ, Ferreira-Nogueira R, Basile-Filho A, et al. Performance of microvesicles as biomarkers of clinical outcome in sepsis and trauma: A pilot study. Biomedicine and Pharmacotherapy. 2022 Feb 1;146. 172. Phetsouphanh C, Darley DR, Wilson DB, Howe A, Munier CML, Patel SK, et al. Immunological dysfunction persists for 8 months following initial mild-to-moderate SARS-CoV-2 infection. Nature Immunology 2022 23:2 [Internet]. 2022 Jan 13 [cited 2023 Jun 14];23(2):210–6. Available from: https://www.nature.com/articles/s41590-021-01113-x 100 173. Bonaventura A, Vecchié A, Dagna L, Martinod K, Dixon DL, Van Tassell BW, et al. Endothelial dysfunction and immunothrombosis as key pathogenic mechanisms in COVID-19. Nature Reviews Immunology 2021 21:5 [Internet]. 2021 Apr 6 [cited 2023 Jun 14];21(5):319–29. Available from: https://www.nature.com/articles/s41577-021-00536-9 174. Guervilly C, Bonifay A, Burtey S, Sabatier F, Cauchois R, Abdili E, et al. Dissemination of extreme levels of extracellular vesicles: tissue factor activity in patients with severe COVID-19. Blood Adv [Internet]. 2021 Feb 2 [cited 2023 Jun 18];5(3):628. Available from: /pmc/articles/PMC7846479/ 175. Rosell A, Havervall S, Von Meijenfeldt F, Hisada Y, Aguilera K, Grover SP, et al. Patients With COVID-19 Have Elevated Levels of Circulating Extracellular Vesicle Tissue Factor Activity That Is Associated With Severity and Mortality—Brief Report. Arterioscler Thromb Vasc Biol [Internet]. 2021 Feb 1 [cited 2023 Jun 18];41(2):878. Available from: /pmc/articles/PMC7837685/ 176. Ware LB. Physiological and biological heterogeneity in COVID-19-associated acute respiratory distress syndrome. Lancet Respir Med [Internet]. 2020 Dec 1 [cited 2023 Jun 19];8(12):1163. Available from: /pmc/articles/PMC7836300/ 177. Gupta A, Madhavan M V., Sehgal K, Nair N, Mahajan S, Sehrawat TS, et al. Extrapulmonary manifestations of COVID-19. Nat Med [Internet]. 2020 Jul 1 [cited 2023 Jun 19];26(7):1017–32. Available from: https://pubmed.ncbi.nlm.nih.gov/32651579/ 178. Oran DP, Topol EJ. Prevalence of Asymptomatic SARS-CoV-2 Infection: A Narrative Review. Ann Intern Med [Internet]. 2020 Sep 1 [cited 2023 Jun 19];173(5):362–8. Available from: /pmc/articles/PMC7281624/ 179. Tarazona-Fernández A, Rauch-Sánchez E, Herrera-Alania O, Galán-Rodas E, Tarazona-Fernández A, Rauch-Sánchez E, et al. ¿Enfermedad prolongada o secuela pos-COVID-19? Acta Médica Peruana [Internet]. 2020 [cited 2023 Jun 20];37(4):565–70. Available from: http://www.scielo.org.pe/scielo.php?script=sci_arttext&pid=S1728-59172020000400565&lng=es&nrm=iso&tlng=es | pt_BR |
dc.publisher.initials | UEA | pt_BR |
Aparece nas coleções: | DISSERTAÇÃO - PPCAH Programa de Pós-Graduação em Ciências Aplicadas à Hematologia |
Arquivos associados a este item:
Arquivo | Descrição | Tamanho | Formato | |
---|---|---|---|---|
Caracterização do perfil de microvesícular circulantes na infecção por SARS-CoV-2 em pacientes e indivíduos convalescentes.pdf | 2,4 MB | Adobe PDF | Visualizar/Abrir |
Os itens no repositório estão protegidos por copyright, com todos os direitos reservados, salvo quando é indicado o contrário.